Quantitative model evaluation of organic carbon oxidation hypotheses for the Ediacaran Shuram carbon isotopic excursion
SHI Wei1, LI Chao1* & Thomas J. ALGEO1,2,3
1State Key Laboratory of Biogeology and Environmental Geology, China University of Geosciences, Wuhan 430074, China;
2State Key Laboratory of Geological Processes and Mineral Resources, China University of Geosciences, Wuhan 430074, China;
3Department of Geology, University of Cincinnati, Cincinnati OH 45221-0013, USA
Received September 10, 2017; accepted November 8, 2017; published online November 21, 2017
Abstract
The largest global carbon-cycle perturbation in Earth history was recorded in the Ediacaran—a persistent negative shift in the global marine dissolved inorganic carbon (DIC) reservoir that lasted for ?25-50 million years, with a nadir of -12%o (i.e., the Shuram Excursion, or SE). This event is considered to have been a result of fUll or partial oxidation of a large dissolved organic carbon (DOC) reservoir, which, if correct, provides evidence for massive DOC storage inthe Ediacaran ocean owing to an intensive microbial carbon pump (MCP). However, this scenario was recently challenged by new hypotheses that relate the SE to oxidization of recycled continentally derived organic carbon or hydrocarbons from marine seeps. In order to test these competing hypotheses, this paper numerically simulates changes in global carbon cycle fluxes and isotopic compositions during the SE, revealing that: (1) given oxygen levels in the Ediacaran atmosphere-ocean of <40% PAL, the recycled continental organic carbon hypothesis and the full oxidation of oceanic DOC reservoir hypothesis are challenged by the atmospheric oxygen availability which would have been depleted in 4 and 6 million years, respectively; (2) the marine-seep hydrocarbon oxidation hypothesis is challenged by the exceedingly large hydrocarbon fluxes required to sustain the SE for >25 Myr; and (3) the heterogeneous (partial) DOC oxidation hypothesis is quantitatively able to account for the SE because the total amount of oxidants needed for partial oxidation (<50%) of the global DOC reservoir could have been met.
Keywords Carbon isotope, Shuram Excursion, Dissolved organic carbon, Atmospheric oxygen, Oceanic oxidation
Citation: Shi W, Li C, Algeo T J. 2017. Quantitative model evaluation of organic carbon oxidation hypotheses for the Ediacaran Shuram carbon isotopic excursion.
Science China Earth Sciences, 60: 2118-2127, https://doi.org/10.1007/s11430-017-9137-1
1.Introduction
The largest negative carbonate carbon isotope (斗3Ccarb) excursion in Earth history (to -12%) is present in the Ediacaran stratigraphic succession (635-541 Ma) that overlies Neoproterozoic glacial diamictites. This event, termed the Shuram Excursion (SE) or Doushantuo Negative Carbon isotope Excursion (DOUNCE), lasted for ?25-50 million years at ?600—550 Ma (Grotzinger et al., 2011; Le Guerroue et al., 2006; Lu et al., 2013) (Figure 1). An unusual feature of the.Corresponding author (email: chaoli@cug.edu.cn)? Science China Press and Springer-Verlag GmbH Germany 2017 SE is that the carbon isotope compositions of organic matter (d13Corg) and the d13Ccarb were decoupled, which deviates from the pattern of ^13Corg-^13Ccarb coupling due to derivation from a common dissolved inorganic carbon (DIC; HCO3VCO32-) reservoir that characterizes the Phanerozoic. This decoupling may have been linked to the existence of a large dissolved organic carbon (DOC) reservoir (>100-1000 x modern level) in the Ediacaran deep ocean with a residence time >10 kyr (Rothman et al., 2003). In this scenario, oxidation of this DOC reservoir generated large amounts of 12C-enriched CO2, resulting in the large decrease in the d13C of oceanic DIC that was recorded by the SE (Rothman et al., 2003; Fike et al., 2006; Jiang et al., 2007, 2011; McFadden et al., 2008; Wangearth.scichina.com link.springer.comet al., 2015; Li et al., 2017). The observed decoupling of *3Corg and *3Ccarb may have been due to buffering ofthe *3C of primary organic carbon in ocean-surface waters by this large DOC reservoir (Rothman et al., 2003; Fike et al., 2006; McFadden et al., 2008). Recently, Li et al. (2017) proposed a spatially heterogenous DOC oxidation hypothesis that attempted to account for the widespread spatial variability of SE records observed within basins and globally. Furthermore, due to the insignificant role of metazoans (which are the main driver of the modern biological pump) in Ediacaran oceanic ecosystems, this large DOC reservoir has been considered as a key line of evidence for an intensive microbial carbon pump (MCP) in the Ediacaran ocean (Jiao et al., 2013, 2014).
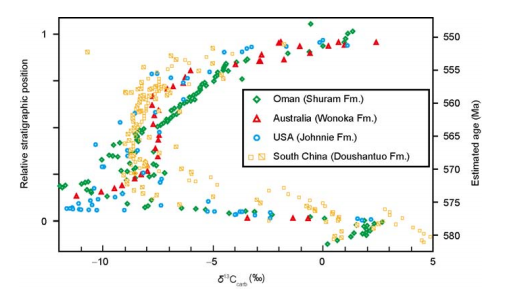
Figure 1 (Color online) Typical records for the largest negative carbon isotope 0"Ccarb) excursion (i.e., Shuram Excursion) in global Ediacaran successions (modified from Grotzinger et al., 2011). Data sources: Oman (Shuram Formation)(Fike et al., 2006); Australia (Wonoka Formation) (Calver et al., 2011); USA (Johnnie Formation) (Verdel et al., 2011); South China (Doushantuo Formation-Jiulongwan section): yellow little square (Li et al., 2017), yellow big square with diagonal (McFadden et al., 2008). Relative stratigraphic position (Grotzinger et al., 2011) and general age framework are based on the Oman Shuram Formation, see the text for details.
The existence of a large DOC reservoir during the Ediacaran was recently challenged. Firstly, the primary nature of the SE signal has been questioned, and alternative origins of the SE linked to diagenetic (e.g., Derry, 2010) or authigenic carbonate processes(e.g., Schrag etal., 2013; Cui et al., 2017) have been proposed. However, these mechanisms are inherently local in operation, which is inconsistent with the global nature ofthe SE (Grotzinger et al., 2011). In addition, these hypotheses are inconsistent with comprehensive evidence from geochemical, mineralogic, petrologic, and stratigraphic studies worldwide (see a review in Li et al., 2017). Secondly, alternative hypotheses have suggested that large amounts of 12C-enriched DIC could have come from oxidation of other forms of organic carbon rather than DOC, e.g., recycled continentally derived organic carbon (Kaufman et al, 2007; Loyd etal., 2013; Osburn et al., 2015) or hydrocarbons from marine seeps (Lee et al., 2015). These non-DOC oxidation hypotheses have challenged the existence of a large DOC reservoir and the prominent role of MCP in the Ediacaran ocean.
To explore the viability of DOC versus non-DOC oxidation hypotheses as the cause of the SE, we conducted a quantitative assessment of these hypotheses through integrated modeling of the oceanic carbon-sulfur cycles. Our quantitative model provides new insights regarding the existence of a large DOC reservoir in late Neoproterozoic oceans, the operation of a MCP, and the origin of the SE.
2.“Six-box model" of the marine carbon-sulfur cycle
Throughout geological history, the marine carbon and sulfur cycles have been closely linked through microbial sulfate reduction (MSR), a paired reaction in which sulfate is reduced to H2S concurrently with oxidation of organic carbon to DIC, yielding energy (Kurtz et al., 2003). This process can cause compositional changes in the atmospheric-oceanic system and attendant variations in climate at time scales of >1 Myr (Kump et al., 1999). Carbon-sulfur cycle linkage is commonly attested by covariation in the isotopic compositions of pyrite sulfur (derived from H2S) and carbonate carbon (derived from DIC). In the anoxic Ediacaran deep ocean, the Fe2+ in pyrite was sourced mainly from hydrothermal vents or submarine volcanoes (Kump and Seyfried, 2005). The linked reactions can be expressed as:

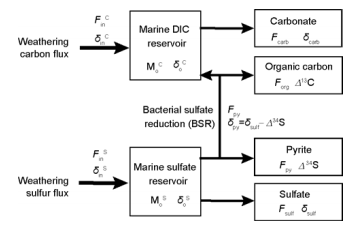
Figure 2 Diagram for “six-box model” of carbon-sulfur cycle (modifiedfrom Kurtz et al., 2003). The symbols for each unit denote the flux (F) ormass (M) and corresponding isotopic compositions ( ) or fractionation ( ).
The marine carbon-sulfur cycles can be represented by a “six-box model" (Figure 2). This model makes use of a sim-plified carbon cycle, in which all forms of carbon in the ocean are assigned to a single reservoir, all carbon inputs to the ocean (including oxidation of organic matter and subaerial weathering of carbonates) are shown as a single source, and all carbon outputs from the ocean (including carbonate precipitation and organic matter burial) as a single sink. Similarly, the sulfur cycle is reduced in the model to a single oceanic reservoir (marine sulfate) having a single source flux (including oxidation of sulfides and subaerial weathering of sulfates) and a single sink flux (including sulfate and pyrite burial). For steady-state C-S cycles, the relationships among the input flux (Fin) and its isotopic composition ,and the output flux (Fout) and its isotopic composition ,may be expressed as

where Mo and 况 denote the mass of the oceanic carbon or sulfur reservoir and its isotopic composition, respectively, f denotes the burial fraction of the reduced phase (i.e., organic carbon or pyrite), and J represents isotopic fractionation between the oceanic reservoir and output fluxes. Because carbon isotope fractionation during carbonate precipitation (l—2%o) is negligible, the M and its isotopic composition 0o) can be expressed as

where Forg and Fcarb are the burial fluxes of marine organic carbon and carbonate, respectively. Eqs. (5) and (6) are also applicable to the steady-state sulfur cycle. However, if there was a large DOC reservoir in the Ediacaran ocean, then the DIC released by its oxidation would have to be treated as a separate carbon source (Fdoc; Tdoc), and the mass of this marine carbon reservoir (MoC) and its isotopic composition 0oC) expressed as

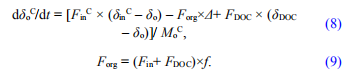
Because the rate of change in the isotopic composition of the marine carbon reservoir is negatively correlated to its mass (i.e., MoC) (eq. (8)), larger values of MoC result in smaller values , resulting in a stronger buffering of its isotopic composition.
Atmosphere oxygen levels are intimately linked to the Earth's carbon and sulfur cycles. Over geologic time, net changes in atmospheric oxygen can be calculated through the differences between burial and weathering fluxes of organic carbon plus pyrite (Kurtz et al., 2003). During photosynthesis, marine primary producers generate one mole of oxygen for each mole of organic carbon produced. In the anoxic and stratified Ediacaran ocean (see Section 3), most organic matter was decomposed by anaerobic organisms, in which two moles of sulfate were required for each 3.5 moles of organic carbon that underwent decay, yielding one mole of pyrite for burial and releasing 3.5 moles of oxygen to the atmosphere (as shown by merging eqs. (1) and (2)). However, pyrite exposed on land was oxidized to sulfate per the following reaction:
4FeS2 + 1502 +8H2O 一 2Fe2O3 + 8SO42-+ 16H+ (10)
where each mole of pyrite is oxidized by 3.75 moles of oxygen. Eqs. (1), (2), and (10) show that, if riverine sulfate were derived entirely from pyrite oxidation, the burial and re-oxidation of each mole of marine pyrite would result in a net decrease of 0.25 moles of atmospheric oxygen. However, riverine sulfate is derived not only from pyrite oxidation but also from sulfate dissolution, and a simple calculation shows that, if the proportion of sulfate flux from pyrite oxidation is lower than 93.3%, a net increase of atmospheric oxygen will occur.
Thus, according to the carbon-sulfur-oxygen biogeochemical cycle described above, whether or not oxygen accumulates in the atmosphere depends on the proportion of pyrite- oxidized sulfate in the total riverine sulfate flux. According to published data compilations, this proportion was 33.3% on average for the Phanerozoic (Kump et al., 1986) (Table 1). Therefore, it is necessary to consider the influence of the sulfur cycle on the carbon-oxygen cycle in our modeling of secular variation in atmospheric oxygen levels and quantitative evaluation of the various organic carbon oxidation hypotheses.
3.Carbon-sulfur cycling in the Ediacaran ocean
Most existing research indicates that, even though the second great oxygenation event (Neoproterozoic Oxygenation Event) occurred during the Ediacaran, atmospheric oxygen concentrations (pO2)were less than 40% of the present atmospheric level (PAL; <15.2^1018 mol), the oceans remained largely anoxic (Lyons et al., 2014; Sperling et al., 2015), and the atmospheric CO2 content (pCO?) was up to 12 x the modern level (Bao et al., 2008). Based on the power relationship between atmospheric CO2 and marine DIC concentrations (Kump et al., 1999), we infer that the DIC reservoir of the Ediacaran ocean may have been ~3 x its average Phanero- zoic size (i.e.,10x1018 mol). In addition, the Ediacaran deep ocean was intensely stratified (e.g., Canfield, 2007; Li et al., 2010, 2015; Johnston et al., 2013; Sahoo et al., 2012, 2016) with mid-depth euxinic waters dynamically coexisting with overlying oxic surface waters and underlying ferruginous deep waters (Li et al., 2010), which is a substantially different redox condition from the dominantly oxic oceans of the Phanerozoic. In the deep Ediacaran ocean, organic matter was oxidized mainly by seawater sulfate rather than dissolved oxygen (Tziperman et al., 2011; Li et al., 2015, 2017). These basic characteristics of the Ediacaran carbon-sulfur cycle are the basis for our mathematical simulation of carbon-sulfur cycling during the SE event.
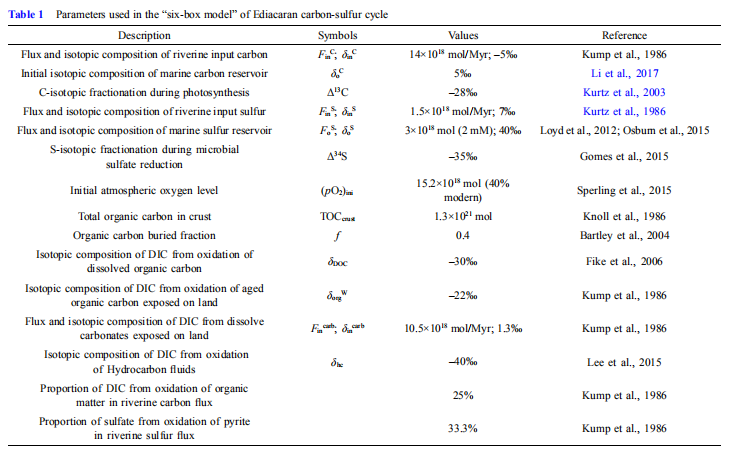
Our quantitative model of the SE carbon-sulfur cycle requires information concerning the size and isotopic composition of each reservoir and the magnitude and isotopic fractionation associated with each flux. Owing to limited knowledge of the Neoproterozoic ocean, we have adopted estimates for some model parameters based on Phanerozoic values (Table 1). The carbon-cycle parameters are mainly from Kump et al. (1986), which avoids uncertainties that might emerge from utilizing multiple sources.
Our mathematical simulation of the Ediacaran marine carbon-sulfur cycle also requires geochronological data for the SE. In South China, the SE started at ~575 Ma, which is 5 million years after the termination of the Gaskiers glaciation (580 Ma) (Bowring et al., 2003). Two zircon U-Pb ages from the bottom and top of the Doushantuo Formation (635.2 0.6 and 551.1 0.7 Ma; Condon et al., 2005) show that it was deposited over an 84-Myr interval. Based on its average deposition rate (~1.4 m/Myr), we estimate that the latter part of the SE (i.e., characterized by a rise in ^13Ccarb from its nadir) dates to 560 Ma (Jiang et al., 2007). This estimate is consistent with a geochronological anchor point of ?560 Ma for the global occurrence of Eoandromeda octobrachi- ata, which has been found in strata in South China dating to the late SE (Zhu et al., 2008). A zircon U-Pb age of 551 Ma from the ash bed at the top of the Doushantuo Formation (Condon et al., 2005) roughly average Phanero- zoic size (i.e.,10x1018 mol). In addition, the Ediacaran deep ocean was intensely stratified (e.g., Canfield, 2007; Li et al., 2010, 2015; Johnston et al., 2013; Sahoo et al., 2012, 2016) with mid-depth euxinic waters dynamically coexisting with overlying oxic surface waters and underlying ferruginous deep waters (Li et al., 2010), which is a substantially different redox condition from the dominantly oxic oceans of the Phanerozoic. In the deep Ediacaran ocean, organic matter was oxidized mainly by seawater sulfate rather than dissolved oxygen (Tziperman et al., 2011; Li et al., 2015, 2017). These basic characteristics of the Ediacaran carbon-sulfur cycle are the basis for our mathematical simulation of carbon-sulfur cycling during the SE event.
Our quantitative model of the SE carbon-sulfur cycle requires information concerning the size and isotopic composition of each reservoir and the magnitude and isotopic fractionation associated with each flux. Owing to limited knowledge of the Neoproterozoic ocean, we have adopted estimates for some model parameters based on Phanerozoic values (Table 1). The carbon-cycle parameters are mainly from Kump et al. (1986), which avoids uncertainties that might emerge from utilizing multiple sources.
Our mathematical simulation of the Ediacaran marine carbon-sulfur cycle also requires geochronological data for the SE. In South China, the SE started at575 Ma, which is 5 million years after the termination of the Gaskiers glaciation (580 Ma) (Bowring et al., 2003). Two zircon U-Pb ages from the bottom and top of the Doushantuo Formation (635.2 0.6 and 551.1 0.7 Ma; Condon et al., 2005) show that it was deposited over an84-Myr interval. Based on its average deposition rate (~1.4 m/Myr), we estimate that the latter part of the SE (i.e., characterized by a rise in ^13Ccarb from its nadir) dates to560 Ma (Jiang et al., 2007). This estimate is consistent with a geochronological anchor point of 560 Ma for the global occurrence of Eoandromeda octobrachi- ata, which has been found in strata in South China dating to the late SE (Zhu et al., 2008). A zircon U-Pb age of 551 Ma from the ash bed at the top of the Doushantuo Formation (Condon et al., 2005) roughly corresponds to the termination of the SE (Figure 1). A nearly identical age range was inferred in Oman, where the SE event began at 580 Ma, i.e., at the unconformity related to Gaskiers glaciation (Bowring et al., 2003; Condon et al., 2005), and terminated at~550 Ma (Fike et al., 2006). For modeling purposes, we set the beginning of the SE at 580 Ma (Oman) or ~575 Ma (South China) and used four anchor points (580, 575, 560, and 550 Ma) for
calculated variables (see abscissas of Figures 3-6).
4.Model Evaluation
4.1 Hypothesis 1: Recycled continental organic carbon oxidation
This hypothesis proposes that the large negative ^13Ccarb excursion of the SE was driven by massive oxidation of recycled continental organic carbon that provided 12C-enriched DIC to the ocean. According to this scenario, enhanced subaerial weathering of old sedimentary organic carbon was triggered by the Pan-African Orogeny, which commenced at 580 Ma (Kaufman et al., 2007; Loyd et al., 2013). A key feature of this hypothesis is that 12C-enriched DIC was generated on land via oxidation by atmospheric oxygen. We evaluate this hypothesis based on the carbon isotope dataset ofthe Jiulong- wan section (South China) reported by Li et al. (2017).
All model scenarios are evaluated in relation to best-fitting linear functional equations for divided intervals of 斗3Ccarb records, which best describe the stratigraphic trend of the SE at study sections. For Hypothesis 1, we divided the SE carbon isotope record of the Jiulongwan Section into three intervals:
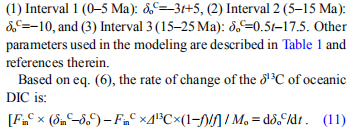
The average riverine DIC input flux during the Phanero- zoic has been 14x1018 mol/Myr, to which carbonate dissolution (Fincarb) contributes 10.5x1018 mol/Myr with an isotopic composition (^incarb) of 1.3% and oxidation of recycled organic matter (Forgw) contributes the remainder (i.e., ~3.5x1018 mol/Myr with a ^incarb of-23.7%). Based on a simple mass balance calculation, the carbon isotopic composition of the total riverine DIC flux 0inC) during the SE can be expressed as

Inserting the best-fitting linear function for each interval and the adopted parameter values into eqs. (11) and (12), we can obtain the recycled continental organic matter weathering flux (i.e., oxygen consumption flux) versus time:
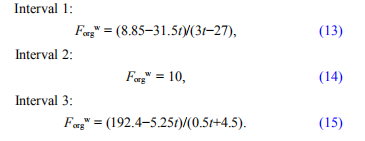
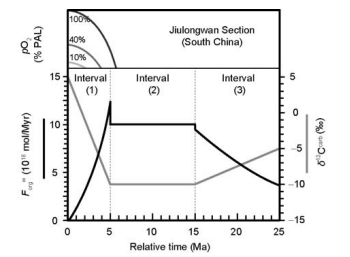
Figure 3 Modeling results of recycled continental organic carbon oxidation hypothesis. The initial atmosphere oxygen level is set to 10%, 40%, and 100% present atmospheric level (PAL), respectively. The carbonate carbon isotope (WCcarb) dataset of Jiulongwan section (South China) for the linear function fitting is from Li et al. (2017); ForgW is ecycled continental organic carbon weathering flux.
The modeling results are shown in Figure 3. Because the sole source ofDIC was from oxidation of recycled continental organic carbon, the oxygen demand is so large that the atmospheric oxygen reservoir would have been exhausted in 4 or 6 Myr given an initial atmospheric oxygen level before the SE of <40% or 100% PAL, respectively. Note: the ongoing O2 contribution by organic burial to the atmospheric oxygen reservoir has been considered in this calculation (see eq. (11)). Therefore, due to limited oxidant supply, the recycled continental organic carbon oxidation hypothesis is difficult to account for the >25 Myr duration of the SE.
4.2 Hypothesis 2: Marine-seep hydrocarbon oxidation
Two additional sources of DIC to the ocean need to be considered for this hypothesis: (1) riverine inputs; (2) oxidation of hydrocarbons released from marine seeps (Lee et al., 2015). In this hypothesis, the formation of the SE is attributed to large inputs of12C-enriched DIC 0hc=-40%o) from heterotrophic degradation (e.g., MSR) ofhydrocarbons from marine seeps (Lee et al., 2015). The amount of hydrocarbons required to generate the SE can be a key test of this hypothesis.
We used the carbon isotope data of the TM-6 drillcore (Oman) reported in Lee et al. (2015) for modeling the organic carbon flux from marine-seep hydrocarbons during the SE. Inserting these equations into eq. (8) yields the following algorithms for carbon fluxes from marine-seep hydrocarbons (Fhc) versus time (t):
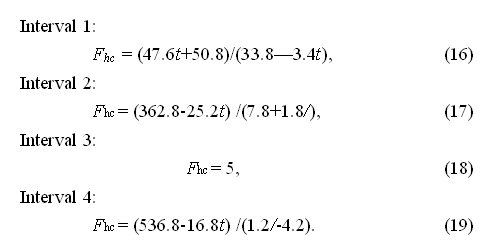
The modeling results are shown in Figure 4. By integrating the mass of organic carbon from hydrocarbons for each interval, the sum for the four intervals is ?2乂1020 mol (6^1015 tons). Although the global crustal organic carbon pool in the Ediacaran is not known, it was likely similar to the modern level (1.3x1021 mol; Des Marais et al., 1992). However, the total amount of hydrocarbon organic carbon (6^1015 tons) required in the hydrocarbon oxidation hypothesis is as much as ~27000 x today's global oil and gas reserves (2.17X1011 tons; Krylov et al., 1997). In addition, based on an oil-expulsion efficiency of ?60% of hydrocarbon-rich source rock (with a potential production of ~5 kg/ton at TOC of ?1.5%), as suggested by Cooles et al. (1986), this total amount of hydrocarbon organic carbon would require ~1.2x1018 tons of source rock. Given the total area of land-ocean on Earth (5x1014 m2) and an average shale density of 2.5 g/cm3, this much source rock would require a 1000-m-thickness of hydrocarbon-rich source rock globally in order to generate sufficient petroleum during the ~25- to 30-Myr interval of the SE. It is exceedingly difficult for these conditions to have been met during the Ediacaran. Therefore, the hydrocarbon oxidation hypothesis is also challenged to account for the SE for >25 Myr due to its exceedingly large hydrocarbon flux demand. It is also worth noting that d13Corg signatures as low as ?40%o (i.e., the average isotopic composition of microbially
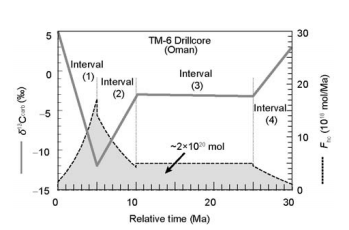
Figure 4 Quantitative modeling results ofhydrocarbon oxidation hypothesis. The carbonate carbon isotope (WCcarb) data of the TM-6 section (Oman) for linear function fitting are from Lee et al. (2015); the shaded area is the sum of the fluxes of organic carbon in marine-seep hydrocarbons (Fhc) for four intervals (~2x1020 mol).
generated hydrocarbons) was not reported from the MQ-1 drillcore by Fike et al. (2006). Therefore, it seems likely that the oxidation of hydrocarbon-bearing fluids was, at most, a local phenomenon in deep-water facies rather than a global event.
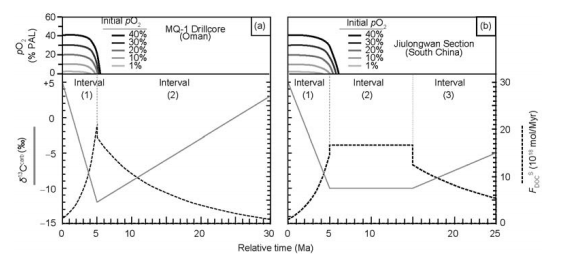
Figure 5 Quantitative modeling results of global ocean DOC reservoir oxidation hypothesis based on carbon-sulfur isotopic datasets of MQ-1 drill in Oman (a) and of Jiulongwan section in South China (b). The initial atmospheric oxygen level (^O2) is set as 1 to 40% of the modern level (PAL) (upper portion of the figure); the carbon-sulfur isotopic data sources: (a) MQ-1 drillcore (Fike et al., 2006); (b) Jiulongwan section (Li et al., 2017); WCcarb: carbonate isotopic composition; Fdocs : sulfate flux for oxidation of the DOC pool.
4.3 Hypothesis 3: Complete oxidation of oceanic DOC reservoir
Rothman et al. (2003) inferred that a large DOC reservoir (?100-1000 x modern level) existed in the Ediacaran ocean. Complete oxidation of this reservoir would have released large amounts of 12C-enriched DIC (-30%), generating a large negative d13Ccarb shift during the SE. A previous study calculated that oxidation of a DOC reservoir of 7x1020 mol (i.e., 6000-12000 x modern level) would be necessary to account for a decline of 〃3Cdic to —12% for a ?30-Myr interval, but that the main oxidant used (e.g., seawater sulfate at 5 mM) would have been exhausted within ?0.8 Myr (Bristow and Kennedy, 2008). This quantitative assessment needs to be revisited. First, the global ^13Ccarb records show large spatial heterogeneity in the magnitude, morphology and timing of the SE (Li et al., 2017). For example, Jiang et al. (2011) reported different SE signals at with different water depths in South China. Second, the SE record in Oman exhibits a decrease of ^13Ccarb from 5 to —12% only during the first 5 Myr of the SE, followed by an increase to 3% for the next ?25 Myr, which is significantly different from the persistently low value of —12% for ~30 Myr assumed by Bristow and Kennedy (2008). As a consequence, the size of the required DOC pool would have been less than 7x1020 mol. Third, riverine sulfate inputs to the Ediacaran ocean were not considered in the model of Bristow and Kennedy (2008).
Additional arguments suggest that it is necessary to re-evaluate the availability of oxidants in the Ediacaran global ocean in order to accurately assess the DOC reservoir oxidation hypothesis. Many lines of evidence support a significant rise of Earth's oxygen levels in the late Neoproterozoic (Neoproterozoic oxidation event or NOE; Lyons et al., 2014). Furthermore, elevated land weathering was also inferred based on global 87Sr/86Sr data during the SE (see a compilation in Li et al., 2017), which may have provided significant amounts of oxidants to the ocean. It is worth noting that carbonate-associated sulfate (CAS) sulfur isotopes (W4Scas) decreased by 20% in the first 5 Myr of the SE, which has been interpreted as a consequence of elevated continental sulfate fluxes lowering the 户S of seawater sulfate after oxidation of the marine DOC reservoir (Fike et al., 2006; McFadden et al., 2008; Li et al., 2017). Therefore, it is necessary for us to re-evaluate the availability of oxidants in the oceanic DOC reservoir oxidation hypothesis.
In this study, we use the C-S isotope datasets of drillcore MQ-1 (Oman) reported in Fike et al. (2006) and the Jiu- longwan section (South China) reported in McFadden et al. (2008) to evaluate the demand of oxidants (O2+SO42-). Based on the carbon-sulfur isotope dataset of the drillcore MQ-1 in Oman and its ?30-Myr duration, the SE can be divided into two intervals:
Interval 1: The d13C ofthe marine DIC pool 0而。)declined from an initial value of 5%o to -12%o over 5 Myr, yielding a ddjdt of -3.4%/Myr. Thus, the best-fitting linear functional equation between》3Cdic 0oC) and time (t) is as follows:
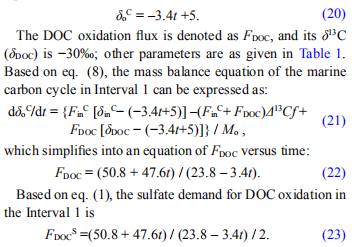
As shown in Figure 5a, the modeling results for the MQ-1 drillcore data demonstrate that the riverine sulfate flux (FinS; equal to Fdocs) needs to increase from ?1.5 1018 mol/Myr (average Phanerozoic level) to ?21 1018 mol/Myr (~14 average Phanerozoic level) over 5 Myr to provide sufficient oxidants. Similar modeling of the Jiulongwan data shows that FinS needs to increase to ~15x1018 mol/Myr (~10 x average Phanerozoic level) over 5 Myr (Figure 5b).
Interval 2: can be given as follows:

Insertion into eq. (8) yields the following relationship between sulfate flux and time for Interval 2:

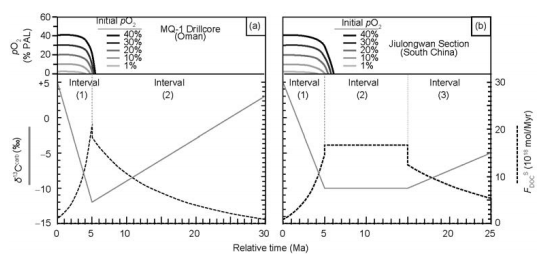
As shown in Figure 5a, the modeling results of the MQ-1 drillcore data demonstrate that riverine sulfate flux (FinS; equal to Fdocs) needs to decrease to ~0.9x1018 mol/Myr over this 25-Myr interval. Similar modeling of the Jiulongwan data (but divided into Intervals 2 and 3) shows that FinS needs to maintain a steady rate of 16.8x1018 mol/Myr for the first 10 Myr (Interval 2) before decreasing to 5.5x1018 mol/Myr during the second 10 Myr (Interval 3; Figure 5b).
Changes in atmospheric oxygen levels (pO?) would have occurred in conjunction with the changes in the Ediacaran carbon-sulfur cycles modeled above. We can estimate the evolution of atmospheric pO? in each of our model scenarios based on the difference between the oxygen released by marine organic carbon and pyrite burial and that consumed by oxidative weathering of sedimentary organic matter and pyrite. Owing to a lack of information about weathering fluxes for the Neoproterozoic, we have adopted the Phanerozoic values for the average fractions of the riverine DIC flux derived from oxidative weathering of organic matter (25%) and of the riverine sulfate flux derived from oxidative weathering of pyrite (33.3%) (Kump et al., 1986). The evolution of atmospheric pO^ is a function of the burial fluxes of marine organic carbon and pyrite [(FinC + Fdoc) x f] as well as the oxidative weathering fluxes of organic matter and pyrite [FinC x 25%+ FinS x 33.3% x (15/8)] (note: 15/8 is the molar ratio between O2 and sulfate during pyrite oxidation; see eq. (10)). The functional equation forpO^ evolution can be given as follows:

The modeling results for the Oman and South China datasets are shown in Figure 5a and 5b, respectively. Our results indicate that atmospheric oxygen would have been exhausted in no more than 6 Myr given an initial atmospheric O2 level of 40% PAL, the maximum estimate for the Ediacaran (Sperling et al., 2015). Note: the ongoing O2 contributions by organic burial to the atmospheric oxygen reservoir and initial oceanic sulfate reservoir have been considered in this calculation (see eqs. (25) and (26)). Therefore, it is highly improbable that the quantities of oxidants (O2 + SO42-) in the Ediacaran atmosphere-ocean were sufficient to permit full oxidation of the oceanic DOC reservoir that existed at that time.
4.4 Hypothesis 4: Heterogeneous (partial) oxidation of oceanic DOC reservoir
The final hypothesis examined here invokes heterogeneous (i.e., partial) oxidation of the oceanic DOC reservoir, as first proposed by Li et al. (2017). This hypothesis is grounded on observations of the significantly heterogeneous character of the SE, e.g., with regard to its magnitude, pattern, and timing, at a global scale. This heterogeneity reflects spatial and temporal variability in the availabilities of DOC and oxidants in different watermasses and/or different basins, resulting in distinctly local characteristics of the SE event. For example, the d13Ccarb record of the SE in the Nanhua Basin of South China exhibits (1) a decline to only 0%o over 5 Myr in the inner-shelf Zhangcunping section due to limited DOC availability; (2) a decline to -12% over 25 Myr in the inner-shelf basinal Jiulongwan section due to high availability of both DOC and oxidants; and (3) a decline to 0% over the first 10 Myr, followed by a further decline to -10% over the next 5 Myr in the upper-slope Siduping section due to dynamic changes in oxidant availability (Li et al., 2017). Spatial heterogeneity of SE records was also reported from coeval strata in Oman and Siberia (e.g., Fike et al., 2006; Lee et al., 2013; Pokrovskii et al., 2006). According to the heterogeneous oxidation hypothesis, large d13Ccarb excursions developed during the SE event only in some specific areas (such as at oceanic mid-depths) rather than in the whole ocean; in other words, the global DOC reservoir was only partially oxidized.
The modeling of heterogeneous (partial) oceanic DOC oxidation proceeded similarly to that for complete oceanic DOC oxidation (Hypothesis 3), but the amounts of DOC and oxidants calculated are based on partial oxidation (10% or 50%) of the marine DOC pool. Our results indicate that, if only 10% of the DOC reservoir is oxidized, the amount of DOC oxidized is ~1.8'1021 mole (equal to ?1500—3000 ' modern level), and atmospheric oxygen levels combined with the continental weathering sulfate flux are sufficient to sustain the SE for its duration of 25 Myr, even if pO2 is only 10% PAL (Figure 6a). Sensitivity testing shows that oxidation of up to 50% of the oceanic DOC reservoir can be sustained for up to 25 Myr with a starting atmospheric pO2 of 40% PAL (Figure 6b). However, if >50% of the oceanic DOC reservoir is oxidized, the supply of oxidants in the Ediacaran atmosphere-ocean system will be insufficient to sustain the SE for 25 Myr (Figure 6b). The average net production of DOC by the microbial carbon pump (MCP) in modern marginal seas is 0.38 Pg C/yr, i.e., 32'1018 mol/Myr (Dai et al., 2016). If we assume a similar level of DOC production in the Ediacaran ocean before the SE, a simple calculation shows that 10% of the Ediacaran oceanic DOC reservoir (~1.8'1021 mol) would have been generated over ~56 Myr, which is roughly equal to the interval between the last Neoproterozoic glaciation (Mari- noan glaciation) and the SE event (?635-575 Ma), supporting the possible accumulation of such a DOC reservoir in the Ediacaran ocean.
5. Conclusions
In this study, we evaluated the viabilities of four hypotheses that have been proposed to account for the Ediacaran Shu- ram Excursion (SE) based on mass-balance modeling. Two of these hypotheses entail oxidation of an oceanic dissolved organic carbon (DOC) reservoir (i.e., the complete oxidation and partial (or heterogenous) oxidation models), and the other two entail oxidation of non-DOC carbon reservoirs (i.e., the recycled continental organic carbon and marine-seep hydrocarbon oxidation models). Given an atmospheric oxygen level of <40% PAL during the Ediacaran, both the recycled continental organic carbon and complete oceanic DOC oxidation hypotheses are challenged by insufficient oxidant supply to sustain oxidation throughout the 25- to 30-Myr-long SE, and the marine-seep hydrocarbon oxidation hypothesis also has difficulties to account for the sustained negative ^13Ccarb shift to -12%o during the SE owing to the demand of the exceedingly large scale ofthe hydrocarbon seeps. Our modeling results indicate that the heterogeneous oceanic DOC oxidation hypothesis, which requires only partial oxidation of the global oceanic DOC reservoir, could have sustained the SE over a 25- to 30-Myr interval with sufficient DOC and oxidant supplies provided that the partial oxidation ratio of the global DOC reservoir remained less than 50%.
Acknowledgements We thank Nianzhi Jiao, Maoyan Zhu, Xuelei Chu, Timothy W. Lyons, Dalton S. Hardisty, Erik A. Sperling, Graham, A. Shields-Zhou, Junhua Huang, Genming Luo, and Chao Li S group for their helpful discussions. We thank the three reviewers for their valuable comments and suggestions. This work was supported by the National Program on Key Basic Research Project (Grant No. 2013CB955704), the National Key Research and Development Program of China (Grant No. 2016YFA0601100), the NSFC-RCUK_NERC Program (Grant No. 41661134048), the Fundamental Research Funds for Central Universities (Grant Nos. CUG-Wuhan; grants 1610491T01 and G1323531767), and the NASA Exobiology Program (TJA).
References
Bao H, Lyons J R, Zhou C. 2008. Triple oxygen isotope evidence for elevated CO2 levels after a Neoproterozoic glaciation. Nature, 453: 504-506
Bartley JK, KahL C. 2004. Marine carbon reservoir, Corg-Ccarb coupling, and the evolution ofthe Proterozoic carbon cycle. Geology, 32: 129-132
Bowring S, Maow P, Landing E, Ramezani J, Grotzinger J. 2003. Geochronological constraints on terminal Neoproterozoic events and the rise of metazoan. Regulat Toxicol Pharmacol, 25: 60-67
Bristow T F, Kennedy M J. 2008. Carbon isotope excursions and the oxidant budget of the Ediacaran atmosphere and ocean. Geology, 36: 863-866
Calver C R. 2000. Isotope stratigraphy of the Ediacarian (Neoproterozoic III) of the Adelaide Rift Complex, Australia, and the overprint of water column stratification. Precambrian Res, 100: 121-150
Canfield D E, Poulton S W, Narbonne G M. 2007. Late-Neoproterozoic deep-ocean oxygenation and the rise of animal life. Science, 315: 92-95 Condon D, Zhu M, Bowring S, Wang W, Yang A, Jin Y. 2005. U-Pb ages from the Neoproterozoic Doushantuo Formation, China. Science, 308: 95-98
Cooles G R Mackenzie A S, Quigley T M. 1986. Calculation of petroleum masses generated and expelled from source rocks. Org Geo Chem, 10: 235-245
Cui H, Kaufman A J, Xiao S, Zhou C, Liu X M. 2017. Was the Ediacaran Shuram Excursion a globally synchronized early diagenetic event? Insights from methane-derived authigenic carbonates in the uppermost Doushantuo Formation, South China. Chem Geol, 450: 59-80
Dai M, Wu K, Meng F. 2016. Net dissolved organic carbon production and carbon partitioning in the marginal seas. Abstracts of the 4th Conference on Earth System Science. Shanghai
Derry L A. 2010. A burial diagenesis origin for the Ediacaran Shuram- Wonoka carbon isotope anomaly. Earth Planet Sci Lett, 294: 152-162
Fike D A, Grotzinger J P, Pratt L M, Summons R E. 2006. Oxidation of the Ediacaran Ocean. Nature, 444: 744-747
Grotzinger J P, Fike D A, Fischer W W. 2011. Enigmatic origin of the largest-known carbon isotope excursion in Earth's history. Nat Geosci, 4: 285-292
Gomes M L, Hurtgen M T. 2015. Sulfur isotope fractionation in modern euxinic systems: Implications for paleoenvironmental reconstructions of paired sulfate-sulfide isotope records. Geochim Cosmochim Acta, 157: 39-55
Jiang G, Kaufman A J, Christie-Blick N, Zhang S, Wu H. 2007. Carbon isotope variability across the Ediacaran Yangtze Platform in South China: Implications for a large surface-to-deep ocean WC gradient. Earth Planet Sci Lett, 261: 303-320
Jiang G, Shi X, Zhang S, Wang Y, Xiao S. 2011. Stratigraphy and paleogeography of the Ediacaran Doushantuo Formation (ca. 635-551 Ma) in South China. Gondwana Res, 19: 831-849
Jiao N Z, Zhang C L, Li C, Wang X X, Dang H Y, Zeng Q L, Zhang R, Zhang Y, Tang K, Zhang Z L, Xu D P. 2013. Controlling mechanisms and climate effects of microbial carbon pump in the ocean (in Chinese). Sci Sin Terrae, 43: 1-18
Jiao N, Robinson C, Azam F, Thomas H, Baltar F, Dang H, Hardman-Mountford N J, Johnson M, Kirchman D L, Koch B P, Legendre L, Li C, Liu J, Luo T, Luo Y W, Mitra A, Romanou A, Tang K, Wang X, Zhang C, Zhang R. 2014. Mechanisms of microbial carbon sequestration in the ocean一Future research directions. Biogeosciences, 11: 5285-5306
Johnston D T, Poulton S W, Tosca N J, O'Brien T, Halverson G P, Schrag D P, Macdonald F A. 2013. Searching for an oxygenation event in the fos- siliferous Ediacaran of northwestern Canada. Chem Geol, 362: 273-286
Kaufman A J, Corsetti F A, Varni M A. 2007. The effect of rising atmospheric oxygen on carbon and sulfur isotope anomalies in the Neoproterozoic Johnnie Formation, Death Valley, USA. Chem Geol, 237: 47-63 DesMarais D J, Strauss H, Summons R E, Hayes J M. 1992. Carbon isotope evidence for the stepwise oxidation of the Proterozoic environment. Nature, 359: 605-609
Krylov N, Mikhaltsev A, Gramberg I, Kouzn O. 1997. Exploration Concepts for the Next Century. Abstract of World Petroleum Congress
Kump L R, Garrels R M. 1986. Modeling atmospheric O2 in the global sedimentary redox cycle. Am J Sci, 286: 337-360
Kump L R, Arthur M A. 1999. Interpreting carbon-isotope excursions: Carbonates and organic matter. Chem Geol, 161: 181-198
Kump L R, Seyfried Jr. W E. 2005. Hydrothermal Fe fluxes during the Precambrian: Effect of low oceanic sulfate concentrations and low hydrostatic pressure on the composition of black smokers. Earth Planet Sci Lett, 235: 654-662
Kurtz A C, Kump L R, Arthur M A, Zachos J C, Paytan A. 2003. Early Cenozoic decoupling of the global carbon and sulfur cycles. Paleoceanography, 18: 1101, doi: 10.1029/2003PA000937
Lee C, Fike D A, Love G D, Sessions A L, Grotzinger J P, Summons R E, Fischer W W. 2013. Carbon isotopes and lipid biomarkers from organic- rich facies of the Shuram Formation, Sultanate of Oman. Geobiology, 11: 406-419
Lee C, Love G D, Fischer W W, Grotzinger J P, Halverson G P. 2015. Marine
organic matter cycling during the Ediacaran Shuram excursion. Geology, 43: 1103-1106
Le Guerroue E, Allen P A, Cozzi A, Etienne J L, Fanning M. 2006. 50 Ma recovery from the largest negative WC excursion inthe Ediacaran ocean. Terra Nova, 18: 147-153
Li C, Planavsky N J, Shi W, Zhang Z, Zhou C, Cheng M, Tarhan L G, Luo G, Xie S. 2015. Ediacaran marine redox heterogeneity and early animal ecosystems. Sci Rep, 5: 17097
Li C, Love G D, Lyons T W, Fike D A, Sessions A L, Chu X. 2010. A stratified redox model for the Ediacaran ocean. Science, 328: 80-83
Li C, Hardisty D S, Luo G, Huang J, Algeo T J, Cheng M, Shi W, An Z, Tong J, Xie S, Jiao N, Lyons T W. 2017. Uncovering the spatial heterogeneity of Ediacaran carbon cycling. Geobiology, 15: 211-224
Loyd S J, Marenco P J, Hagadorn J W, Lyons T W, Kaufman A J, Sour-Tovar F, Corsetti F A. 2012. Sustained low marine sulfate concentrations from the Neoproterozoic to the Cambrian: Insights from carbonates of northwestern Mexico and eastern California. Earth Planet Sci Lett, 339-340: 79-94
Loyd S J, Marenco P J, Hagadorn J W, Lyons T W, Kaufman A J, Sour-Tovar F, Corsetti F A. 2013. Local d34S variability in ?580 Ma carbonates of northwestern Mexico and the Neoproterozoic marine sulfate reservoir. Precambrian Res, 224: 551-569
Lu M, Zhu M, Zhang J, Shields-Zhou G, Li G, Zhao F, Zhao X, Zhao M. 2013. The DOUNCE event at the top of the Ediacaran Doushantuo Formation, South China: Broad stratigraphic occurrence and non-diagenetic origin. Precambrian Res, 225: 86-109
Lyons T W, Reinhard C T, Planavsky N J. 2014. The rise of oxygen in Earth's early ocean and atmosphere. Nature, 506: 307-315
McFadden K A, Huang J, Chu X, Jiang G, Kaufman A J, Zhou C, Yuan X, Xiao S. 2008. Pulsed oxidation and biological evolution in the Ediacaran Doushantuo Formation. Proc Natl Acad Sci USA, 105: 3197-3202
Osburn M R, Owens J, Bergmann K D, Lyons T W, Grotzinger J P. 2015. Dynamic changes in sulfate sulfur isotopes preceding the Ediacaran Shu- ram Excursion. Geochim Cosmochim Acta, 170: 204-224
Pokrovskii B G, Melezhik V A, Bujakaite M I. 2006. Carbon, oxygen, strontium, and sulfur isotopic compositions in late Precambrian rocks of the Patom Complex, central Siberia: Communication 1. results, isotope stratigraphy, and dating problems. Lithol Miner Resour, 41: 450-474
Rothman D H, Hayes J M, Summons R E. 2003. Dynamics of the Neoproterozoic carbon cycle. Proc Natl Acad Sci USA, 100: 8124-8129
Sahoo S K, Planavsky N J, Jiang G, Kendall B, Owens J D, Wang X, Shi X, Anbar A D, Lyons T W. 2016. Oceanic oxygenation events in the anoxic Ediacaran ocean. Geobiology, 14: 457-468
Sahoo S K, Planavsky N J, Kendall B, Wang X, Shi X, Scott C, Anbar A D, Lyons T W, Jiang G. 2012. Ocean oxygenation in the wake of the Marinoan glaciation. Nature, 489: 546-549
Schrag D P Higgins J A, Macdonald F A, Johnston D T. 2013. Authigenic carbonate and the history of the global carbon cycle. Science, 339: 540-543
Sperling E A, Wolock C J, Morgan A S, Gill B C, Kunzmann M, Halverson G P, Macdonald F A, Knoll A H, Johnston D T. 2015. Statistical analysis of iron geochemical data suggests limited late Proterozoic oxygenation. Nature, 523: 451-454
Tziperman E, Halevy I, Johnston D T, Knoll AH, Schrag D P. 2011. Biologically induced initiation of Neoproterozoic snowball-Earth events. Proc Natl Acad Sci USA, 108: 15091-15096
Verdel C, Wernicke B P, Bowring S A. 2011. The Shuram and subsequent Ediacaran carbon isotope excursions from southwest Laurentia, and implications for environmental stability during the metazoan radiation. Geolog Soc Am Bull, 123: 1539-1559
Wang H, Li C, Hu C, Xie S. 2015. Spurious thermoluminescence characteristics of the Ediacaran Doushantuo Formation (ca. 635-551 Ma) and its implications for marine dissolved organic carbon reservoir. J Earth Sci-China, 26: 883-892
Zhu M, Gehling J G, Xiao S, Zhao Y, Droser M L. 2008. Eight-armed Edi- acara fossil preserved in contrasting taphonomic windows from China and Australia. Geology, 36: 867-870