Carbon sequestration processes and mechanisms in coastal mariculture environments in China
ZHANG YongYu1,2*, ZHANG JiHong3, LIANG YanTao1, LI HongMei1, LI Gang1,2,CHEN Xiao4, ZHAO Peng5, JIANG ZengJie3, ZOU DingHui6, LIU XiaoYong7 & LIU JiHua2,4
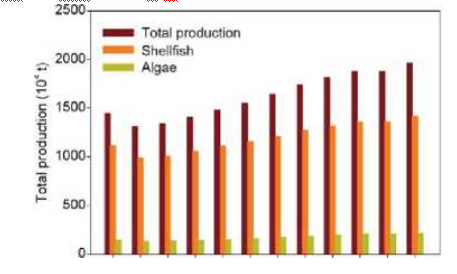
1 Key Laboratory of Biofuels, Shandong Provincial Key Laboratory of Energy Genetics, Qingdao Institute of Bioenergy and Bioprocess Technology,Chinese Academy of Sciences, Qingdao 266101, China; 2 Joint Laboratory of Microbial Oceanography, Qingdao National Laboratory for Marine Science and Technology, Qingdao 266237, China; 3 Yellow Sea Fisheries Research Institute, Chinese Academy of Fishery Sciences, Qingdao 266071, China; 4 Institute of Marine Science and Technology, Shandong University, Qingdao 266273, China; 5 National Marine Data and Information Service, Tianjin 300171, China; 6 School of Environment and Energy, South China University of Technology, Guangzhou 510006, China; 7 Shandong Haizhibao Marine Sci. & Tech. Ltd. Co., Weihai 264300, China
Received October 21, 2017; accepted November 16, 2017; published online November 21, 2017
Abstract China is the global leader in mariculture production. Increasing sequestered marine carbon (also known as blue carbon) via mariculture activities is a promising approach for mitigating climate change and promoting the development of a low-carbon economy. Mariculture blue carbon is also considered an important component of China's “sea granary”. In addition to shellfish and macroalgae yields, which represent carbon removed from mariculture environment, blue carbon also includes other important components, which have been largely neglected in the past, such as the carbon transformed by microbes, dissolved organic carbon (mainly referred to as recalcitrant dissolved organic carbon), and sedimentary particulate carbon. Hence, from different aspects, a comprehensive study on the formation processes and mechanisms of carbon sequestration is of great significance for comprehensively unveiling the carbon sequestration capability in coastal mariculture environment, which will contribute to the sustainable development of the fishery economy and construction of an ecological civilization. Moreover, it may add significant economic benefits to the future carbon-trading market.
Keywords Macroalgae and shellfish mariculture, Marine carbon sequestration, Microbe, Recalcitrant dissolved organic carbon, Carbon sedimentation
Citation: Zhang Y Y, Zhang J H, Liang Y T, Li H M, Li G, Chen X, Zhao P, Jiang Z J, Zou D H, Liu X Y, Liu J H. 2017. Carbon sequestration processes and
mechanisms in coastal mariculture environments in China. Science China Earth Sciences, 60: 2097-2107, https://doi.org/10.1007/s11430-017-9148-7
1.Introduction
As the country with the highest CO2 emissions worldwide, China is now accelerating the development of a low-carbon economy and controlling carbon emissions to cope with climate change. In recent years, the growth ofChina's economy has gradually slowed down after a long period of stunning increase. In such situations, establishing an effective mechanism for enhancing marine carbon sequestration and exploring pilot projects for carbon sequestration in ocean ecosystems have become an integral part of the “Integrated Reform Plan for Promoting Ecological Progress” and the “Work Plan to Control Greenhouse Gas Emissions during the 13th Five-Year Plan period”. Recently, Chinese scientists have established the Pan-China Ocean Carbon Alliance (COCA) and China Future Ocean Alliance (C-FOA) and launched the
earth.scichina.com link.springer.com
“China Blue Carbon Initiative". The oceans are the largest carbon pool on earth and play an important role in regulating global climate changes. China is surrounded by 4.7 million km2 of pericontinental sea, of which approximately 3 million square kilometers is under the direct control of China. Development of effective approaches to increase carbon sinks is promising based on in-depth studies of marine carbon sequestration processes and mechanisms. At present, the known mechanisms of ocean carbon sequestration mainly include the solubility pump and biological pump (BP). Because the solubility pump is mainly controlled by natural processes, its capability of enhancing carbon sequestration through human control is limited; whereas the BP which is mainly driven by marine organisms, has great potential for exploitation, owing to the controllability of biological organisms. BP and microbial carbon pump (MCP) are the two most important biologically driven carbon sequestration mechanisms known to date. The BP is a process of transport and storage of biogenic particles from surface water to deeper water based on a series of biological processes, including phytoplanktonic CO2 fixation, consumption, transport, sedimentation, and decomposition (Longhurst and Harrison, 1989). The MCP is a process independent of particulate carbon deposition and involves the generation of recalcitrant dissolved organic matter (RDOM) and long-term carbon storage (more than five thousand years) mainly driven by microbial metabolism (Ogawa and Tanoue, 2003; Jiao et al., 2010). As a key regulator of the marine carbon cycle, the MCP was called “the invisible hand behind a vast carbon reservoir" (Stone, 2010). Many technological improvements have been implemented for increasing carbon sequestration based on the research on BP and MCP mechanism, such as the strategy of a carbon sequestration fishery (Tang, 2014; Tang and Liu, 2016) and the strategy of increasing carbon sequestration via integrative management of the land-ocean system (Jiao et al., 2011c). These strategies provided new scientific approaches to blue carbon exploration.
China boasts the highest mariculture production worldwide. The implementation of biological carbon sequestra- tion/carbon storage, including the exploration of a carbon sequestration fishery, plays a very important role in coping with climate change and in developing a low-carbon economy. The initially recognized carbon sink mechanisms in mariculture environment involve the processes of promoting carbon dioxide fixation via fishery activities and removing this carbon from the water column (removable carbon). However, recent research has provided a considerably deeper and comprehensive understanding of the formation processes and mechanisms of carbon sequestration in coastal mariculture environment. In addition to the removable carbon in mariculture environment (e.g., shellfish/kelp aquaculture products), the dissolved organic carbon (DOC) and recalcitrant dissolved organic carbon (RDOC) driven by microbes, as well as particulate organic carbon (POC) deposition, are also important parts of the sequestered carbon in mariculture environment. However, in the past, there was no standard calculation method to evaluate this blue carbon (carbon sequestered in water) owing to the difficulties in studying these invisible carbon components. Thus, many of the carbon sequestration processes and pathways were neglected in the past. Recent efforts in blue carbon sequestration studies have revealed that these neglected carbon sequestration processes and pathways contribute to a large proportion of blue carbon.
In this paper, the analysis of the significance of carbon sequestration in coastal mariculture environment is presented, and the research progress on the formation processes and mechanisms of carbon sequestration in mariculture environment is summarized. In addition, the future development of the mariculture carbon sequestration industry is prospected.
2.Significance of mariculture blue carbon development
Climate change is a serious threat to the sustainable development of the world. Previous research indicates that reducing carbon emissions and increasing carbon sinks are the two foremost ways to deal with climate change. Currently, China is the largest carbon dioxide emitter in the world. As mentioned in “Enhanced Actions on Climate Change: China's Intended Nationally Determined Contributions", China promised that carbon emissions would peak in 2030 and a carbon trading market would be fully launched in 2017, in order to promote the development of a low-carbon economy and carbon sink industry. However, because the current national economic growth rate has been slowing down, relying solely on emission reduction may further aggravate the negative impact on the national economy. Thus, in addition to emission reduction, enhancing carbon storage from the atmosphere is a good approach. As is known, the ocean is the largest carbon pool on Earth and plays an important role in regulating climate change via absorbing CO2. More than half of the global carbon captured through photosynthesis is captured by marine life, and that portion is called blue carbon, or blue carbon sinks (Nelleman et al., 2009). Globally distributed vegetation habitats, such as mangroves, salt marshes, and seagrass beds, are important coastal carbon sinks. Marine mariculture organisms, phytoplankton, microorganisms, etc. are also important contributors to blue carbon sinks. Refractory dissolved organic carbon (RDOC), which is mainly formed through microbial biotransformation, as well as sedimentary organic carbon, comprise the long-term stored carbon pool of the ocean.
At present, China is actively leading the world in coping with climate change. Considering the great potential of the oceans in dealing with and mitigating climate change, in “sion for Maritime Cooperation under the Belt and Road Initia-tive", which was jointly released in June 2017 by the State Oceanic Administration and the National Development and Reform Commission, the goals of increasing marine carbon sinks and achieving green development in the fUture were highlighted, and “Strengthening cooperation in addressing climate change" and “Strengthening international blue carbon cooperation" were emphasized.
China has the largest mariculture industry worldwide. To meet the new challenges of the current conditions and of world economic development, combining the mariculture carbon sequestration with the sea granary construction is a newly explored scientific development approach in recent years. It is a win-win strategy to increase coastal carbon sequestration and promote the development of a low-carbon economy. Specific implementation approaches include the following aspects: improvement of the water quality of coastal mariculture environment, restoration and enhancement of the carbon capturing capability of algae (such as restoration of seagrass beds), and mariculture mode adjustment to enhance carbon sink capability (for example, by encouraging the construction of ecological pasture-like seaweed farms). Mariculture blue carbon exploitation is an important practice of the Chinese government to cope with and mitigate climate change. It is also imperative that the sustainable development of a marine ecological civilization and fishery economy be promoted. In the future, blue carbon is expected to become a valuable and tradable commodity in the national and international carbon markets. The exploration of carbon sequestration mechanisms and blue carbon assessment in mariculture environment is of great importance to provide theoretical and technical support in the future carbon market. The development of mariculture blue carbon is expected to become a promising new economic growth point.
3.Status and function in carbon sequestration of Chinese mariculture
China is the world's largest mariculture country, in terms of both mariculture area and total output. Since the 1960s, mariculture production has been continuously increasing (Figure 1). The total mariculture output of China reached 19.6 million tons in 2016, which is 42% higher than in 2005 (Fishery Administration of the Ministry of Agriculture, 2006-2017). By 2014, China's total mariculture production accounted for 62% of the world's output (FAO, 2016). The dominant cultured organisms in China are shellfish and macroalgae, which together account for more than 85% of China's marine mariculture production (Fishery Administration of the Ministry of Agriculture, 2006-2017). Macroalgae, or seaweed, can convert dissolved inorganic carbon and CO2 into organic carbon via photosynthesis (Li et al., 2007; Zou and Gao, 2014; JietYear
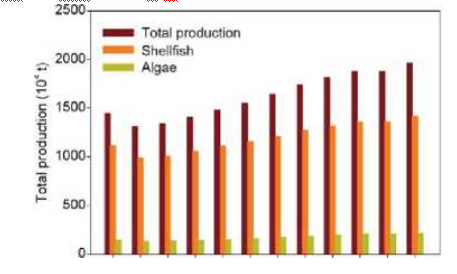
Figure 1 Total production of coastal macroalgae and shellfish mariculture in China for 2005-2016 (104 tons).
al., 2016), and the nitrate and phosphate dissolved in seawater can be absorbed during their growth (Xu etal., 2010; Xu et al., 2011; Xu and Gao, 2012). All ofthese cause the alkalinity of seawater to rise continuously, which decreases the partial pressure of CO2 in seawater, thus promoting the absorption of CO2 by seawater, which is the basis for ocean carbon fixation functioning. Shellfish also play an important role in the marine carbon cycle by removing particulate organic carbon from seawater through feeding and the forming of shells with calcium carbonate as the main component.
The fixed carbon in marine aquaculture is mainly in four forms: carbon fixed in cultured organisms that can be removed from the seawater, known as the removable carbon; particulate organic carbon (POC) and dissolved organic carbon (DOC) stored in seawater (Ji and Wang, 2015), as well as the buried carbon in sediments. Figure 2 shows the carbon sequestration and transport pathways in macroalgae (e.g., kelp) mariculture environment.
Many scientists worldwide have carried out much research on the estimation and assessment of the carbon sequestration potential in mariculture environment, but in the past, the emphasis has been on the removable carbon of shellfish and macroalgae. Employing the Chinese mariculture statistical data from 2005 to 2012 as a reference, the annual average carbon fixation capability of mariculture in China was assessed at over 1 million tons of carbon (Ji and Wang, 2015). Seaweed farming plays an important role in mariculture carbon fixation. For example, it is estimated that 700 million tons of carbon (0.7 Gt) can be fixed per year by macroalgae in the global continental shelf (He et al., 2015). Among the diverse macroalgae in China, kelp plays a major role in mariculture carbon fixation, contributing about 73% of all the fixed carbon of macroalgae mariculture. Wakame, nori, and Gracilaria are some of the other cultivated macroalgae. The yield of these four macroalgae types accounted for more than 97% of the total macroalgae mariculture output in China (Ji and Wang, 2015).
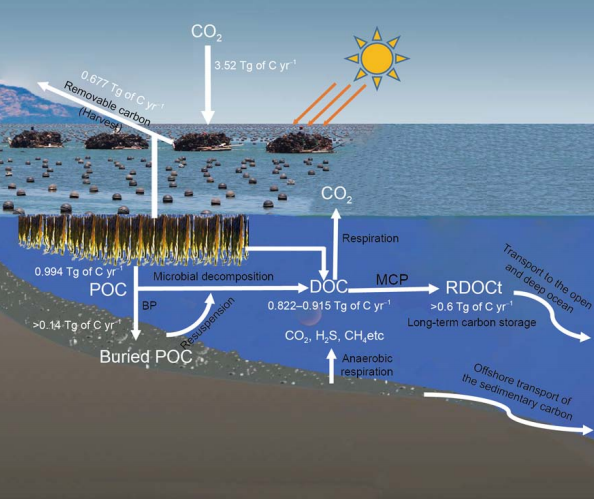
Figure 2 Diagram of the carbon sequestration and transport pathways in macroalgae (e.g., kelp) mariculture environment. Based on the total production of macroalgae mariculture of China in2016, the primary production ofmacroalgae (mainly kelp) was calculated as ca. 3.52 Tg of C, the removable organic carbon as ca. 0.677 Tg of C, the released DOC as ca. 0.822-0.915 Tg ofC, the exported RDOCt as greater than 0.6TgofC,the formed POC as ca. 0.994 Tg of C, and the deposited carbon as greater than 0.14 Tg of C. MCP, microbial carbon pump; BP, biological carbon pump; RDOCt, environmental context-specific RDOC.
In fact, except for the removable carbon, coastal mariculture contributes to carbon sequestration functioning by producing marine dissolved carbon (including RDOC), particulate organic carbon, and sedimentary carbon. Microbes, including phytoplankton, bacteria, viruses, and protists, that are inhabiting mariculture environment also play important roles in driving carbon sequestration and are considered important components of mariculture blue carbon. For example, phytoplankton, which is at the base ofthe food web, is a major contributor to the primary productivity of the oceans. The organic carbon produced by phytoplankton is transferred through the food web to higher-level consumers, such as fish and shellfish. According to the carbon ecological conversion efficiency, the carbon fixed through phytoplankton photosynthesis is eventually dispersed into various components of mariculture blue carbon (Sun, 2013). As an important component of the microbial food web, microbes are important drivers of the marine biogeochemical cycle and exercise carbon sequestration functions. Nowadays, under the negative impacts of global warming, ocean acidification, and coastal eutrophication, the water quality of mariculture environment shows a declining trend. Sometimes even hypoxia can be formed, which causes serious impact to mariculture activities. In such conditions, as compared with the cultivated organisms, microbes will play increasingly important roles in driving the marine carbon cycle and become significant contributors to blue carbon.
Large amounts of DOC and POC can be released into the seawater during the process of mariculture. Some of this organic carbon is rapidly utilized by microorganisms and transformed into biomass, and then transported through the microbial food loop and food chain to different trophic levels, such as protozoa, zooplankton, fish, and shrimp, to eventually become a part of the removable carbon. A portion of the unutilized POC (including microorganism or algae debris, small organic carbon polymers, and animal and plant residues) can sink to the seafloor and constitute the important components of deposited carbon stocks in the mariculture environment. In addition, considerable portions of DOC and POC are converted into CO2 through microbial respiration and thus returnto the atmosphere. Meanwhile, microbial metabolic activities via the “microbial carbon pump" (MCP) mechanism can drive biotransformation of labile DOC to recalcitrant DOC (RDOC). This portion of carbon is not rapidly mineralized, can be stored for a long time in seawater, and can form the stable RDOC pool originating from the mariculture environment.
4.Processes, mechanisms, and capabilities of carbon sequestration in coastal mariculture environment
The formation processes of mariculture blue carbon are complex and need interdisciplinary research, covering physical oceanography, biological oceanography, atmospheric science, etc. To date, it is well known that both algae and shellfish mariculture activities can remove carbon from seawater and promote carbon fixation. In addition to this, the processes of carbon sequestration in mariculture environment also involve multiple aspects including CO2 exchange fluxes at the sea-air interface, carbon transformation by microorganisms, formation of DOC and POC pools, and carbon sedimentation (Figure 2). These include not only carbon fixation and storage but also carbon release. Therefore, a comprehensive and in-depth understanding of the key processes and influential parameters of carbon sequestration in mariculture environment is a prerequisite and the basis for a scientific, objective, and reasonable assessment of mariculture blue carbon.
4.1 Removable carbon of macroalgae and shellfish mariculture environment
The removable carbon of shellfish and algae aquaculture mainly refers to the harvested carbon of macroalgae, shellfish, and other cultivated products. The removable algal carbon is formed mainly through growth via photosynthetic carbon fixation. The removable shellfish carbon refers mainly to the formation of calcium carbonate shells during growth. At present, the calculation of the removable carbon amount is based on the carbon content and yields of dried organisms. For 1999 to 2008, the annual removable carbon from macroalgae mariculture in China was 0.3-0.38 (on average 0.34) million tons. In those 10 years, 3.42 million tons of C was removed from seawater via macroalgae mariculture activities. The annual removable carbon in the form of shells from shellfish mariculture was 0.7-0.99 (on average 0.67) million tons. In those 10 years, 8.62 million tons of carbon was removed from seawater. In other words, from 1999 to 2008, 1-1.37 (on average 1.2) million tons of carbon was removed from seawater via macroalgae and shellfish mariculture activities, which is equivalent to removing 4.4 million tons of CO2 per year. If measured according to the algorithm of forestry carbon, the annual contribution to the reduction in atmospheric CO2 by macroalgae and shellfish mariculture activities in China was equivalent to that by the afforestation of more than 0.5 million hectares of trees (Zhang etal., 2005; Tang etal., 2011). Song (2011)estimated that about 4.5 million tons of carbon could be fixed via cultivating economic algae in China each year. If the output of macroalgae mariculture is increasing by 5% annually, the annual carbon fixation by macroalgae mariculture can reach 0.93 million tons of C by 2020.
With the improvement of scientific recognition and assessment techniques, the produced POC and DOC in macroalgae mariculture environment began being widely recognized. Taking the macroalgae yield and the formation of POC and DOC into consideration, Yan et al. (2011) estimated that the total amounts of carbon fixation by macroalgae in China and the world were 783800 tons and 1272500 tons of C per year, respectively.
The carbon sequestration function of shellfish is still controversial. The main reason is that shellfish respiration and calcification can result in CO2 emissions. The carbon sequestration function of shellfish should be considered from the entire aquaculture system. There are two ways for shellfish to store carbon in the ocean. One is to absorb the HCO3- (bicarbonate) in the seawater to form CaCO3 (shell constituent), and its equation is as follows: Ca2++2HCO^=CaCO 3+CO2+H 2O. Although when every 1 mol of calcium carbonate is formed, 1 mol of CO2 is released, but 2 mol of hydrogen carbonate can be absorbed from seawater. The other way is to promote the growth of shellfish soft tissues by filtering the particulate organic carbon (including phytoplankton and particulate organic debris) in water. The amount of carbon emitted during the formation of shellfish soft tissues and the different treatments and processing of post-harvest soft tissues are important parameters in assessing the carbon sequestration function. At the same time, the sedimentation of fecal and pseudo fecal sediments formed during the lifespan of shellfish can accelerate the vertical transport of carbon and promote the formation of a depositional carbon pool. From 1999 to 2014, 0.90 0.10 million tons of C each year was removed from the seawater by shellfish mariculture, of which 0.69 0.06 million tons was the carbon fixed in shells.
To enhance carbon sequestration capability, it is an effective strategy to set a reasonable shellfish and macroalgae proportion in integrated mariculture environment. The macroalgae can absorb nitrate, phosphate, and carbon dioxide released from shellfish, and shellfish can absorb different detrital POC produced by macroalgae. Shellfish and macroalgae interact and support each other, which enhances the carbon sequestration function of the integrated mariculture system. Tang et al. (2013) conducted a comparative study on the amount of carbon sequestration betweento the atmosphere. Meanwhile, microbial metabolic activities via the “microbial carbon pump" (MCP) mechanism can drive biotransformation of labile DOC to recalcitrant DOC (RDOC). This portion of carbon is not rapidly mineralized, can be stored for a long time in seawater, and can form the stable RDOC pool originating from the mariculture environment.
4. Processes, mechanisms, and capabilities of carbon sequestration in coastal mariculture environment
The formation processes of mariculture blue carbon are complex and need interdisciplinary research, covering physical oceanography, biological oceanography, atmospheric science, etc. To date, it is well known that both algae and shellfish mariculture activities can remove carbon from seawater and promote carbon fixation. In addition to this, the processes of carbon sequestration in mariculture environment also involve multiple aspects including CO2 exchange fluxes at the sea-air interface, carbon transformation by microorganisms, formation of DOC and POC pools, and carbon sedimentation (Figure 2). These include not only carbon fixation and storage but also carbon release. Therefore, a comprehensive and in-depth understanding of the key processes and influential parameters of carbon sequestration in mariculture environment is a prerequisite and the basis for a scientific, objective, and reasonable assessment of mariculture blue carbon.
4.1 Removable carbon of macroalgae and shellfish mariculture environment
The removable carbon of shellfish and algae aquaculture mainly refers to the harvested carbon of macroalgae, shellfish, and other cultivated products. The removable algal carbon is formed mainly through growth via photosynthetic carbon fixation. The removable shellfish carbon refers mainly to the formation of calcium carbonate shells during growth. At present, the calculation of the removable carbon amount is based on the carbon content and yields of dried organisms. For 1999 to 2008, the annual removable carbon from macroalgae mariculture in China was 0.3-0.38 (on average 0.34) million tons. In those 10 years, 3.42 million tons of C was removed from seawater via macroalgae mariculture activities. The annual removable carbon in the form of shells from shellfish mariculture was 0.7-0.99 (on average 0.67) million tons. In those 10 years, 8.62 million tons of carbon was removed from seawater. In other words, from 1999 to 2008, 1-1.37 (on average 1.2) million tons of carbon was removed from seawater via macroalgae and shellfish mariculture activities, which is equivalent to removing 4.4 million tons of CO2 per year. If measured according to the algorithm of forestry carbon, the annual contribution to the reduction in atmospheric CO2 by macroalgae and shellfish mariculture activities in China was equivalent to that by the afforestation of more than 0.5 million hectares of trees (Zhang etal., 2005; Tang etal., 2011). Song (2011) estimated that about 4.5 million tons of carbon could be fixed via cultivating economic algae in China each year. If the output of macroalgae mariculture is increasing by 5% annually, the annual carbon fixation by macroalgae mariculture can reach 0.93 million tons of C by 2020.
With the improvement of scientific recognition and assessment techniques, the produced POC and DOC in macroalgae mariculture environment began being widely recognized. Taking the macroalgae yield and the formation of POC and DOC into consideration, Yan et al. (2011) estimated that the total amounts of carbon fixation by macroalgae in China and the world were 783800 tons and 1272500 tons of C per year, respectively.
The carbon sequestration function of shellfish is still controversial. The main reason is that shellfish respiration and calcification can result in CO2 emissions. The carbon sequestration function of shellfish should be considered from the entire aquaculture system. There are two ways for shellfish to store carbon in the ocean. One is to absorb the HCO3- (bicarbonate) in the seawater to form CaCO3 (shell constituent), and its equation is as follows: Ca2++2HCO^=CaCO 3+CO2+H 2O. Although when every 1 mol of calcium carbonate is formed, 1 mol of CO2 is released, but 2 mol of hydrogen carbonate can be absorbed from seawater. The other way is to promote the growth of shellfish soft tissues by filtering the particulate organic carbon (including phytoplankton and particulate organic debris) in water. The amount of carbon emitted during the formation of shellfish soft tissues and the different treatments and processing of post-harvest soft tissues are important parameters in assessing the carbon sequestration function. At the same time, the sedimentation of fecal and pseudo fecal sediments formed during the lifespan of shellfish can accelerate the vertical transport of carbon and promote the formation of a depositional carbon pool. From 1999 to 2014, 0.90 0.10 million tons of C each year was removed from the seawater by shellfish mariculture, of which 0.69 0.06 million tons was the carbon fixed in shells.
To enhance carbon sequestration capability, it is an effective strategy to set a reasonable shellfish and macroalgae proportion in integrated mariculture environment. The macroalgae can absorb nitrate, phosphate, and carbon dioxide released from shellfish, and shellfish can absorb different detrital POC produced by macroalgae. Shellfish and macroalgae interact and support each other, which enhances the carbon sequestration function of the integrated mariculture system. Tang et al. (2013) conducted a comparative study on the amount of carbon sequestration betweenalso plays an important role in the coastal carbon cycle. Filter-feeding shellfish can remove part of the POC in seawater by feeding, which promotes the further transfer and transformation of carbon in the food chain. In addition, shellfish produce a large amount of feces, which can not only accumulate atthe bottom ofthe shallow sea, but also release DOC into the seawater. At present, the contribution of shellfish to the carbon cycle is not understood. However, recent studies have shown that the DOC released by shellfish (such as oysters and scallops) can be very quickly absorbed and utilized again. In other words, the DOC released from shellfish mariculture cannot be easily accumulated in seawater and contributes little to the long-term DOC pool in the ocean. In contrast, the DOC released by macroalgae, e.g., kelp, is more refractory and can be accumulated in seawater, thus the macroalgae mariculture zone shows a higher DOC yield as compared to the non-mariculture zone (Mahmood et al., 2017).
4.4 Carbon sequestration driven by microbes
Marine microbes mainly refer to nanoplankton and picoplankton, which have diameters of less than 20 and 2 pm, respectively. They include various eukaryotic and prokaryotic unicellular organisms. These microbes are considered the main drivers of marine element cycling and energy flow. Marine phytoplankton produce, through photosynthesis, about 50% of the O2 needed for human respiration and fix a similar proportion of CO2 in the ocean (Dubilier et al., 2015). In recent years, extensive studies on marine carbon biogeochemical cycles have greatly increased our knowledge regarding the processes and mechanisms of biologically driven carbon sequestration. For example, with the MCP theory, the microbial carbon transformation effect was systematically elaborated and the source of the huge RDOC pool in the ocean was interpreted. The MCP enriches our understanding of the biologically driven carbon sequestration mechanisms. Now we recognize that carbon fixation is not equal to carbon sequestration in the ocean (Jiao et al., 2010, 2014; Jiao, 2012). In mariculture environment, phytoplankton absorb CO2 from the atmosphere through photosynthesis to synthetize biomass, presenting themselves directly or indirectly as food for fish and shellfish. This is beneficial for the reproduction and maintenance of marine fishery resources. Therefore, the microbial blue carbon can eventually be distributed to different levels of mariculture blue carbon. For example, phytoplankton is the main food source of filter-feeding shellfishes, and the removable carbon, such as shellfish, is essentially from the fixed carbon of marine microbes, such as phytoplankton. Based on this, the fishery carbon sink is ever considered an important branch of the phytoplankton-driven carbon sink (Sun, 2013). Moreover, in mariculture environment, microbes, such as bacteria, are seawater “scavengers" that can degrade and remove pollutants (such as petroleum, pesticides, and herbicides) and are beneficial to the protection and sustainable utilization of marine fishery resources (Radwan et al., 2002). More importantly, the microbes themselves are the main drivers of carbon sequestration function in the ocean. Although they are small, they are the most abundant and widely distributed organisms in the ocean. The total microbial biomass accounts for as much as 90% of the total biomass in the ocean (Pomeroy et al., 2007) and contributes greater than 60% of the total oceanic primary productivity (>29.1 Gt) (Uitz et al., 2010). Even in the continental shelf waters (e.g., southern Yellow Sea) and estuaries (e.g., Pearl River estuary), microbes can contribute more than 50% to the total primary production (Huang et al., 2005; Fu et al., 2009). In recent decades, the coastal water quality has declined significantly. Eutrophication, red/green tides, hypoxia, etc. have occurred frequently in coastal environment, which has negatively influenced the quality and output of mariculture products and could weaken the carbon sequestration potential of coastal waters. In those unfavorable environments, the microbial contributions to the mariculture blue carbon are more prominent.
Moreover, microbes and macroalgae are usually inseparable. The DOC and POC released by macroalgae are important carbon sources supporting microbial growth. Microbial growth processes convert organic matter into inorganic nutrients and trace elements, which greatly affects the photosynthetic growth of macroalgae. In addition, the cultivated animals can release large amounts of organic carbon during their growth. This released organic carbon is highly labile and can be returned to the atmosphere easily via microbial respiration, allowing the carbon to stay in the ocean only for a short time. This cannot be considered a fraction of real marine carbon sequestration. However, the labile organic carbon in mariculture environment can be rapidly absorbed and utilized by microbes and undergoes a series of metabolic and transformational processes to form RDOC. The RDOC can be stored in seawater for as long as thousands of years (Jiao etal., 2010, 2015). It is reported that 95% of the marine DOC pool is RDOC that is not bioavailable (Hansell et al., 2009; Jiao et al., 2011a, 2015). The RDOC pool with its long-term carbon storage capacity is an important fraction of the mariculture blue carbon, and microbes are the main contributors to the formation of the RDOC pool (Jiao et al., 2010, 2011b, 2014). Microbes drive carbon sequestration through a series of metabolic activities and form a huge marine organic carbon pool, of which about 97% exists in the form of DOC (Hansell et al., 2009). The key formation processes of carbon sequestration include microbial-driven carbon fixation and generation or release of RDOC (Zhang et al., 2017). The contribution of the MCP to the global oceanic carbon storage can reach 0.2 Pg C yr-1, which is comparable to that of the BP (Legendre et al., 2015). In macroalgae mariculture environment, the production of RDOCt driven by the MCP is almost equivalent to the removable carbon of macroalgae, which is greater than 0.6 million tons of C per year. In short, the biomass of microorganisms, such as phytoplankton and bacteria, is an important part of the blue carbon in mariculture environment. The DOC (mainly refers to RDOC) pool generated mainly via the MCP is the invisible blue carbon making important contributions to the carbon sequestration in mariculture environment.
4.5 Carbon sedimentation
The buried organic carbon in coastal sediments is one of the most important components of the ocean's vast carbon stocks. Coastal mariculture activities contribute significantly to carbon sedimentation (Krause-Jensen and Duarte, 2016). The biological pump is an important theoretical basis for the development of fishery carbon sequestration. The processes involve the precipitation or sink of POC from the surface into the deep waters. Although in the open oceanic environment, most of the POC is degraded and remineralized to CO2 during the sinking process, little POC can enter the sediments, resulting in limited outputs of POC to the deep ocean. However, in the shallow continental shelf area, carbon sedimentation is very high. The BP-induced carbon deposition forms an important fraction of the buried POC in the shelf sediments. Based on the detritus production rate of kelp forests (706 g C m-2 yr-1 on average) (Krumhansl and Scheibling, 2012) and the macroalgae mariculture area in China in 2016, it is estimated that the released POC from macroalgae mariculture is about 0.994 million tons of C per year. In addition, kelp roots and partial stems usually remain on the rafts after harvest and fall into the seawater later, becoming part of the POC. Hence, the released POC in macroalgae mariculture might be higher than 0.994 million tons of C per year. A portion of this POC is converted to DOC through microbial degradation, and the rest, which is not completely degraded, could sink to the seafloor. Beside this, in the macroalgae and shellfish mariculture environment, the fecal pellets of the shellfish containing undigested algal and other organic detritus can also constitute organic carbon deposits on the seafloor. The carbon reaching the sediments is mostly buried in the seabed, excluding the parts that are resuspended in the seawater and further decomposed by microbes. In the Chlamys fiarreri mariculture zone of Sanggou Bay, it was reported that about 8.71乂104 tons of C per year could be deposited via biodeposition at the water-sediment interface. Most of this carbon is buried and separated from aquatic biogeochemical cycles (Zhang et al., 2011). In kelp mariculture zone, the POM sedimentation rate was about 273-279 g m-2 day-1 from May to July; whereas, after harvest, from October to November, the sedimentation rate had decreased significantly, to 189 g m-2 d-1. The average POM sedimentation rate in different periods was about 162.34 g m-2 day-1 (Cai et al., 2003). According to the average POM sedimentation rate, the organic carbon content (0.17-0.87%) in sediment (Xia et al., 2014), and the total macroalgae mariculture area in China, it is estimated that the organic carbon deposition flux in Chinese macroalgae mariculture environment is above 0.14 million tons ofC per year. This shows that, apart from the removable carbon, carbon sedimentation contributes greatly to mariculture carbon sequestration. Meanwhile, some of the deposited organic carbon can be further transported into the open and deep ocean, driven by ocean currents and other physical processes (Figure 2).
4.6 Carbon release driven by microbial respiration
For a comprehensive assessment of the carbon sequestration processes in mariculture environment, in addition to the various carbon fixation and carbon storage pathways, the carbon release processes should also be considered. It is well known that microbial respiration is a key factor in driving carbon release in the global oceans, especially in the eutrophic coastal environments. The high-density macroalgae and shellfish mariculture activities in coastal environments can weaken water exchange between the inside and outside zone of the mariculture environment. Thus, the organic carbon concentration inside the mariculture area is usually much higher than that of adjacent non-mariculture areas. In addition, large amounts of shellfish fecal pellets and algal debris with rich organic matter will be deposited on the seafloor (Xia et al., 2013). In this environment, with extremely high organic carbon concentration, microbial metabolism is vigorous and can remineralize large quantities of organic carbon into CO2 and release it into the atmosphere. Furthermore, in the sediments with overly rich organic matter, it is easy for hypoxia or anaerobic conditions to form, driven by microbial anaerobic respiration, which can induce the release of CH4, CO2, SO2, etc. This is not only harmful for the cultured organisms, but also weakens the carbon sequestration capability of the mariculture environment. A study in Jiaozhou Bay showed that the CH4 released in mariculture environments varied in different seasons, but the sediment was always the net source of CH4 during the entire year. The annual flux of CH4 release from sediment to seawater in Jiaozhou Bay is about (1.0-1.74)^107 mol per year (Yang, 2009). Microbial metabolism during anaerobic methanogenic activity in sediment is an important driving force for the conversion of organic carbon to CH4. Carbon release driven by microbial respiration can offset carbon sequestration to a certain extent in both mariculture water and sediment. At present, quantitative studies on CO2 release from mariculture environment are scarce and need to be strengthened.
4.7 Carbon transport to the deep and open ocean
Currently, macroalgae and shellfish mariculture is mainly distributed in the shallow shelf sea with depth of less than 30-50 m. In the Yellow Sea, Bohai Sea, and East China Sea, the mariculture activities are especially intensive. In these mariculture environments, the DOC and POC concentrations in seawater, the sediment thickness, and the burial organic carbon content in sediments are all much higher than that in the surrounding area and the open ocean. However, the complicated hydrodynamic conditions in the continental shelf, such as ocean circulation, tidal currents, and waves, can induce the resuspension of sedimentary carbon and the long-distance transport of organic carbon to the open and deep ocean (Shi et al., 2016; Qiao et al., 2017). For example, in recent years, terrigenous organic matter has been found, even in the sediments of Okinawa Trough at the edge of the continental shelf of the East China Sea and in the Mariana Trench, at deeper than 7000 meters (Qiao et al., 2017; Luo et al., 2017). As an important part of the blue carbon in mariculture environment, DOC, RDOC, and sedimentary carbon would be transported to the deep sea to achieve long-term storage in the oceans under the influences of complex hydrodynamic factors. It is estimated that 90% of the total annual carbon storage of macroalgae forests can be transported to the deep ocean (Krause-Jensen and Duarte, 2016). This organic carbon that is transported long distance to the deep sea is also an invisible part of the mariculture blue carbon.
5.Controversy on carbon sequestration in coastal macroalgae and shellfish mariculture
Currently, the definition of carbon sequestration by macroalgae mariculture is still controversial internationally, mainly because the annual plants usually are not listed as carbon sinks by the international society. Macroalgae mariculture is an annual activity. Traditionally, the macroalgae blue carbon mainly refers to the annually harvested algal carbon biomass, or the removable carbon. The focus of the controversy is how long this removable carbon can be stored out of the atmosphere. Indeed, for the removable carbon of cultured macroalgae, the different treatments or processing of the harvested algae have a major impact on the carbon storage time. If the macroalgae material is used for food, it will very soon be reconverted into CO2 and released back to the atmosphere, unable to form a long-term carbon sink. However, if it is used to produce biofuels, it can reduce to a certain extent the carbon emissions caused by burning fossil fuels, which can be considered another form of “emission reduction". The reason why the annual macroalgae were not included in the list of carbon sequestration organisms in the past is that the list only focused on the carbon biomass of macroalgae, and ignored the release of large amounts of organic carbon into the ocean. A large portion of this released organic carbon can sink to the sediment or be transformed into RDOC and thus stored in the ocean for a long time. The total carbon of RDOC is equivalent to the harvested carbon biomass. Meanwhile, a considerable portion of sedimentary carbon can be transported to the deep and open ocean. In fact, the production of RDOC and the carbon sedimentation both contribute greatly to the carbon sequestration in macroalgae mariculture environment, and should be considered indisputably as the real carbon sequestration processes.
Moreover, the view on carbon sequestration of shellfish mariculture is also controversial, primarily because shellfish respiration and calcification can release carbon dioxide. During the shell calcification process, the formation of 1 mol of CaCO3 will be accompanied by the release of 1 mol of CO2, and 2 mol of hydrogen carbonate can be absorbed from seawater. The formation of shells is equivalent to removing 1 mol of carbon from the unstable inorganic carbon pool in the seawater and converting it into stable carbon.
6.Conclusion
Coastal mariculture is one of the important scientific approaches for coping with global climate change and promotes sustainable development in a low-carbon economy. The development of coastal mariculture blue carbon has become a part of the agricultural modernization construction in China. In-depth studies on the carbon sequestration processes and mechanisms in mariculture environment are important in achieving this goal. At present, the importance of mariculture activities for enhancing coastal carbon sequestration is recognized and assessed; meanwhile, some important strategies to increase carbon sinks have been put forward, such as promoting the construction of ecological pastures mainly with seaweed cultivation and accelerating the ecological restoration of seagrass bed ecosystems. Indeed, the carbon storage in mariculture environment includes not only the removable carbon biomass, but also the invisible carbon, such as RDOC, sedimentary carbon, and carbon transported to the open and deep ocean. In the future, with the development of the international and domestic carbon trading management system, blue carbon will most likely be included in the future carbon market as a tradable good. Similar to green carbon, the development of mariculture blue carbon may promote the emergence of a new economic growth point. It is important to promote the sustainable development of coastal mariculture activities by uncovering the key carbon cycling processes and mechanisms in mariculture environment, setting up evaluation methods and techniques for scientifically assessing mariculture blue carbon, and optimizing the mariculture modes considering both environmental and economicbenefits.
Acknowledgements We thank Prof Jiao NianZhi from Xiamen University for providing valuable advice and suggestions. We thank the two anonymous reviewers for their valuable comments and suggestions. This work was supported by the National Key Research and Development Program of China (Grant No. 2016YFA0601402), the Open Task of Qingdao National Laboratory for Marine Science and Technology (Grant No. QNLM2016ORP0311), and the Key R&D Project in Shandong Province (GrantNo. 2015GSF115036).
References
Abdullah M I, Fredriksen S. 2004. Production, respiration and exudation of dissolved organic matter by the kelp Laminaria hyperborea along the west coast of Norway. J Mar Biol Ass, 84: 887-894
Cai L S, Fang J G, Liang X M. 2003. Natural sedimentation in large-scale aquaculture areas of Sango Bay, north China Sea (in Chinese). J Fish Sci China, 10: 305-310
Cao F J. 2017. Study on the bacterial production, the utilization of dissolved organic carbon and the control factors in the South China Sea. Dissertation for Master Degree. Xiamen: Xiamen University
Dubilier N, McFall-Ngai M, Zhao L. 2015. Microbiology: Create a global microbiome effort. Nature, 526: 631-634
FAO. 2016. The State of World Fisheries and Aquaculture 2016. Contributing to Food Security and Nutrition for All. Rome: Food and Agriculture Organization. 200
Fishery Bureau ofMinistry of Agriculture of the People's Republic of China. 2016. China Fisheries Statistics Yearbook. Beijing: China Agriculture Press
Fu M Z, Wang Z L, Sun P, Li Y, Li R X. 2009. Size structure and potential export of phytoplankton primary production in the southern Huanghai (Yellow) Sea (in Chinese). Acta Oceanol Sin, 31: 100-109
Gan S, Wu Y, Zhang J. 2016. Bioavailability of dissolved organic carbon linked with the regional carbon cycle in the East China Sea. Deep-Sea Res Part II-Top Stud Oceanogr, 124: 19-28
Hansell D A, Carlson C A, Repeta D J, Schlitzer R. 2009. Dissolved organic matter in the ocean: A controversy stimulates new insights. Oceanography, 22: 202-211
He P M, Liu Y Y, Zhang JW,WuHL,YuKF, Huo Y Z, Zhang J H. 2015. Research progress on the effects of macroalgae on carbon sink (in Chinese). J Fish Sci China, 22: 588-595
Huang B Q, Hong H S, Ke L, Cao Z Y. 2005. Size-fractionated phytoplankton biomass and productivity in the Zhujiang River Estuary in China (in Chinese). Acta Oceanol Sin, 27: 180-186
Ji J Y, Wang PP. 2015. Research on China's mariculture carbon sink capacity and influencing factors (in Chinese). Mar Environ Sci, 34: 871-878
Ji Y, Xu Z, Zou D, Gao K. 2016. Ecophysiological responses of marine macroalgae to climate change factors. J Appl Phycol, 28: 2953-2967
Jiang Z J, Fang J G, Han T T, Li J Q, Mao Y Z, Wang W. 2013. Estimation of sea-air CO2 flux in seaweed aquaculture area, Lidao Bay (in Chinese). Prog Fish Sci, 34: 50-56
Jiang Z J, Fang J G, Wang W, Li J Q, Meng W, Tan L T, Hou S Y. 2012. Sea-air CO2 flux in Crassostrea gigas aquaculture area of East Gongjia Island, Rushan, Shandong, in autumn. J Fish China, 36: 1592-1598
Jiang Z, Li J, Qiao X, Wang G, Bian D, Jiang X, Liu Y, Huang D, Wang W, Fang J. 2015. The budget of dissolved inorganic carbon in the shellfish and seaweed integrated mariculture area of Sanggou Bay, Shandong, China. Aquaculture, 446: 167-174
Jiao N Z. 2012. Carbon fixation and sequestration in the ocean, with special reference to the microbial carbon pump (in Chinese). Sci Sin Terr, 42:1473-1486
Jiao N Z, Herndl G J, Hansell D A, Benner R, Kattner G, Wilhelm S W, KirchmanDL, WeinbauerM G, Luo T, Chen F, Azam F. 2010. Microbial production of recalcitrant dissolved organic matter: Long-term carbon storage in the global ocean. Nat Rev Microbiol, 8: 593-599
Jiao N Z, Herndl G J, Hansell D A, Benner R, Kattner G, Wilhelm S W, Kirchman D L, Weinbauer M G, Luo T, Chen F, Azam F. 2011a. The microbial carbon pump and the oceanic recalcitrant dissolved organic matter pool. Nat Rev Microbiol, 9: 555-555
Jiao N Z, Legendre L, Robinson C, Thomas H, Luo Y W, Dang H, Liu J, Zhang R, Tang K, Luo T W, Li C, Wang X X, Zhang C L. 2015. Comment on “Dilution limits dissolved organic carbon utilization in the deep ocean". Science, 350: 1483-1483
Jiao N Z, Luo T W, Zhang Y, Zhang R, Tang K, Chen F, Zeng Y H, Zhang Y Y, Zhao Y L, Zheng Q, Li Y L. 2011b. Microbial carbon pump in the ocean-from microbial ecological process to carbon cycle mechanism (in Chinese). J Xiamen Univ-Nat Sci, 50: 387-401
Jiao N Z, Robinson C, Azam F, Thomas H, Baltar F, Dang H, Hardman-Mountford N J, Johnson M, Kirchman D L, Koch B P, Legendre L, Li C, Liu J, Luo T, Luo Y W, Mitra A, Romanou A, Tang K, Wang X, Zhang C, Zhang R. 2014. Mechanisms of microbial carbon sequestration in the ocean一Future research directions. Biogeosciences, 11: 5285-5306
Jiao N Z, Tang K, Cai H Y, Mao Y J. 2011c. Increasing the microbial carbon sink in the sea by reducing chemical fertilization on the land. Nat Rev Microbiol, 9: 75-75
Krause-Jensen D, Duarte C M. 2016. Substantial role of macroalgae in marine carbon sequestration. Nat Geosci, 9: 737-742
Krumhansl K, Scheibling R. 2012. Production and fate of kelp detritus. Mar Ecol Prog Ser, 467: 281-302
Legendre L, Rivkin R B, Weinbauer M G, Guidi L, Uitz J. 2015. The microbial carbon pump concept: Potential biogeochemical significance in the globally changing ocean. Prog Oceanogr, 134: 432-450
Li J Y, Murauchi Y, Ichinomiya M, Agatsuma Y, Taniguchi K. 2007. Seasonal changes in photosynthesis and nutrient uptake in Laminaria japon- ica (Laminariaceae; Phaeophyta). Aquac Sci, 55: 587-597
Liu W Y, Zhang S Y, Wang K, Chen Q M. 2011. Ecology study of the relationship between heterotrophic bacterioplankton and ecological environment in the area of Gouqi Island in spring (in Chinese). J Shanghai Ocean Univ, 20: 437-444
Longhurst A R, Glen Harrison W. 1989. The biological pump: Profiles of plankton production and consumption in the upper ocean. Prog Oceanogr, 22: 47-123
Lu Z M, Dai M H. 2006. Advances in Air-Sea CO2 flux study and the application of eddy covariance technique (in Chinese). Adv Earth Sci, 21: 1046-1057
Luo M, Gieskes J, Chen L, Shi X, Chen D. 2017. Provenances, distribution, and accumulation of organic matter in the southern Mariana Trench rim and slope: Implication for carbon cycle and burial in hadal trenches. Mar Geol, 386: 98-106
Mahmood T, Fang J, Jiang Z, Ying W, Zhang J. 2017. Seasonal distribution, sources and sink of dissolved organic carbon in integrated aquaculture system in coastal waters. Aquacult Int, 25: 71-85
Mann K H. 2000. Ecology of Coastal Waters: With Implications for Management. Oxford: Blackwell Science
Nelleman C, Corcoran E, Duarte C M, Valdes L, Deyoung C, Fonseca L, Grimsditch G. 2009. Blue carbon: The role of healthy oceans in binding carbon. Rev Bras Cienc Solo, 32: 589-598
Ogawa H, Tanoue E. 2003. Dissolved organic matter in oceanic waters. J Oceanogr, 59: 129-147
Ogawa H, Amagai Y, Koike I, Kaiser K, Benner R. 2001. Production of refractory dissolved organic matter by bacteria. Science, 292: 917-920
Pomeroy L, leB. Williams P, Azam F, Hobbie J. 2007. The microbial loop. Oceanography, 20: 28-33
Qiao L L, Wang Z, Liu S D, Li G X, Liu X, Huang L L, Xue W J, Zhong Y. 2017. From continental shelf seas to the western Pacific: The path and
mechanism of cross-shelf suspended sediment transport in the Yellow Sea and East China Sea (in Chinese). Earth Sci Front, 24: 134-140
Radwan S S, Al-Hasan R H, Salamah S, Al-Dabbous S. 2002. Bioremediation of oily sea water by bacteria immobilized in biofilms coating macroalgae. Int Biodeter Biodegr, 50: 55-59
Sabine C L, Feely R A, Gruber N, Key R M, Lee K, Bullister J L, Wanninkhof R, Wong C S, Wallace DWR, Tilbrook B, Millero F J, Peng T H, Kozyr A, Ono T, Rios A F. 2004. The oceanic sink for anthropogenic CO2. Science, 305: 367-371
Shi X F, Hu L M, Qiao S Q, Bai Y Z. 2016. Progress in research of sedimentary organic carbon in the East China Sea: Sources, dispersal and sequestration (in Chinese). Adv Mar Sci, 34: 313-327
Song J M. 2011. Carbon cycling processes and carbon fixed by organisms in China marginal seas (in Chinese). J Fish Sci China, 18: 703-711
Stone R. 2010. The invisible hand behind a vast carbon reservoir. Science, 328: 1476-1477
Sun J. 2013. Carbon calculation on marine phytoplankton and its related fishery carbon sink (in Chinese). Prog Fish Sci, 34: 90-96
Takahashi T, Sutherland S C, Sweeney C, Poisson A, Metzl N, Tilbrook B, Bates N, WanninkhofR, Feely R A, Sabine C, Olafsson J, Nojiri Y. 2002. Global sea-air CO2 flux based on climatological surface oceanpCO2, and seasonal biological and temperature effects. Deep-Sea Res Part II-Top Stud Oceanogr, 49: 1601-1622
Tang Q S. 2014. Management strategies of marine food resources under multiple stressors with particular reference of the Yellow Sea large marine ecosystem. Front Agr Sci Eng, 1: 85-90
Tang Q S, Fang J G, Zhang J H, Jiang Z J, Liu H M. 2013. Impacts of multiple stressors coastal ocean ecosystems and integrated multi-trophic aquaculture (in Chinese). Prog Fish Sci, 34: 1-11
Tang Q S, Liu H. 2016.Strategy for carbon sink and its amplification in marine fisheries (in Chinese). Eng Sci, 18: 68-73
Tang Q S, Zhang J H, Fang J G. 2011. Shellfish and seaweed mariculture increase atmospheric CO2 absorption by coastal ecosystems. Mar Ecol Prog Ser, 424: 97-104
Uitz J, Claustre H, Gentili B, Stramski D. 2010. Phytoplankton class-specific primary production in the world's oceans: Seasonal and interannual variability from satellite observations. Glob Biogeochem Cycle, 24: GB3016-1696
Voros L, V-Balogh K, Koncz E, Kovacs A. 2003. Phytoplankton and bacterioplankton production in a reed-covered water body. Aquatic Bot, 77:
99-110
Whittaker R H. 1975. Communities and Ecosystems. 2nd ed. New York: MacMillan Publ. Co
Xia B, Chen B J, Cui Y, Cui Z G, Zhang X Z, Liu H D, Ding D S, Jiang Z J. 2013. Distribution and source of organic carbon in Sanggou Bay in summer (in Chinese). Prog Fish Sci, 34: 44-49
Xia B, Cui Y, Chen B, Cui Z, Qu K, Ma F. 2014. Carbon and nitrogen isotopes analysis and sources of organic matter in surface sediments from the Sanggou Bay and its adjacent areas, China. Acta Oceanol Sin, 33: 48-57
Xu D, Gao Z, Zhang X, Qi Z, Meng C, Zhuang Z, Ye N. 2011. Evaluation of the potential role of the macroalga Laminaria japonica for alleviating coastal eutrophication. Bioresource Tech, 102: 9912-9918
Xu Z, Gao K. 2012. NH4+ enrichment and UV radiation interact to affect the photosynthesis and nitrogen uptake of Gracilaria lemaneiformis (Rhodophyta). Mar Pollut Bull, 64: 99-105
Xu Z G, Zou D G, Gao K S. 2010. Effects of elevated CO2 and phosphorus supply on growth, photosynthesis and nutrient uptake in the marine macroalga Gracilaria lemaneiformis (Rhodophyta). Botanica Marina, 53: 123-129
Yan L W, Huang H J, Chen J T, Yang X G. 2011. Estimation of carbon sink capacity of algal mariculture in the coastal areas of China (in Chinese). Adv Mar Sci, 29: 537-545
Yang J. 2009. Biogeochemistry of Methane and Nitrous oxide in the water and sediments of Jiaozhou Bay. Dissertation for Master Degree. Qingdao: Ocean University of China
Zhang J H, Fang J G, Tang Q S. 2005. The contribution of shellfish and seaweed mariculture in China to the carbon cycle of coastal ecosystem (in Chinese). Adv Earth Sci, 20: 359-365
Zhang M L, Zou J, Mao Y Z, Zhang J H, Fang J G. 2011. Contribution of culturing scallop to carbon cycle in Sanggou Bay (in Chinese). Fish Moderniz, 38: 13-16
Zhang Y, Zhao M X, Cui Q, Fan W, Qi J G, Chen Y, Zhang Y Y, Gao K S, Fan J F, Wang G Y, Yan C L, Lu H L, Luo Y W, Zhang Z L, Zheng Q, Xiao W, Jiao N Z. 2017. Processes of coastal ecosystem carbon sequestration and approaches for increasing carbon sink. Sci China Earth Sci, 60: 809-820
Zou D, Gao K. 2014. Temperature response of photosynthetic light- and carbon-use characteristics in the red seaweed Gracilariopsis lemaneiformis (Gracilariales, Rhodophyta). J Phycol, 50: 366-375