The shift of biogeochemical cycles indicative of the progressive marine ecosystem collapse across the Permian-Triassic boundary: An analog to modern oceans
State Key Laboratory of Biogeology and Environmental Geology, China University of Geosciences, Wuhan 430074, China
Received November 3, 2017; revised March 23, 2018; accepted April 17, 2018; published online May 29, 2018
Abstract
Global warming, the most severe faunal mass extinction and the shift of biogeochemical cycles were observed in the ocean across the Permian-Triassic boundary about 252 million years ago, providing an analog to understanding the modern oceans. Along with the progressive global warming, the biogeochemical cycle was documented to show a shift from the decoupled processes of carbon, nitrogen and sulfur prior to the mass extinction to the coupled biogeochemical processes during faunal mass extinction. The coupled biogeochemical cycle was further observed to shift from the coupled C-N processes during the first episode of the faunal mass extinction to the coupled C-N-S processes during the second episode, diagnostic of the progressive development of more deteriorated marine environmental conditions and the more severe biotic crisis across the Permian-Triassic boundary. The biogeochemical cycles could thus be an indication to the progressive collapse of marine ecosystems triggered by the global warming in Earth history. In modern oceans, the coupled C-N cycle triggered by the global warming was observed in some regions. If these local C-N processes develop and expand to the global oceans, the coupled C-N-S processes might be brought into existence and the marine ecosystems are inevitable to suffer from complete collapse as observed at 252 million years ago.
Keywords Microbes, Mass extinction, Permian-Triassic boundary, Euxinia, Global warming, Geobiology
1.Introduction
The ocean system is experiencing great changes, including sea surface temperature rising, sea water acidification, marginal sea eutrophication, expansion of the regional minimum oxygen zones, marine biodiversity decrease and coral bleaching, etc. Due to the accelerated decrease in biodiversity, it is thought Earth might suffer or be suffering from the sixth mass extinction (Barnosky et al., 2011), though highly debated. It is a great challenge to decipher the cause and effect relationship among these complicated processes, which is the basis to predict the future trend of the oceanic variations. In particular, the impact of these changes on oceanic ecosystems is very hard to predict due to the large uncertainties of the chemical, physical and biotic processes in modern days and in the future.
Notably, all these changes being occurred in modern oceans were documented to occur in Earth history for many times (Xie and Yin, 2014; Xie et al., 2017a), with some being associated with the collapse of marine ecosystems. The ancient oceans could thus be an analog to the modern oceans, and to deciphering the complicated oceanic processes. It is well known that great changes took place across the Permian-Triassic boundary about 252 million years ago, including the shift of icehouse to greenhouse climate, the most severe mass extinction in Phanerozoic and the extensive volcanisms. After about 5 million years, the ocean ecosystems collapsed could be fully recovered at the end of Early Triassic. Deciphering the pattern and causes of the marine ecosystem collapse across the Permian-Triassic boundary is of great significance, which favors our understanding of the impact of global warming on the ecosystems in modern days, helps us to cope with the co-development of human beings and the natural environments, and provides an early warning on the potential risk of the great disasters in future Earth.
2.Episodic collapse of marine ecosystems associated with temperature rises
It is known that over 90% marine species are believed to go extinct across the Permian-Triassic boundary (Erwin, 2006), including radiolarians, foraminifers, brachiopods, rugose corals, ammonoids, conodonts, sponges, bryozoans, cri- noids, trilobites, etc. (Jin et al., 2000). On the basis of faunal stratigraphical distribution, microbial records and the associated environmental conditions at Meishan GSSP section (Xie et al., 2005, Yin et al., 2012), the mass extinction was proposed to occur as a pattern of two episodes (Figure 1). The two-episodic pattern was late confirmed by the compiled data of 17 genera and 537 species of marine ecosystems collected from 7 sites in South China (Song et al., 2013). The first episode was documented to occur at the conodont zone of Clarkina meishanensis at the end-Permian, featured by the disappearance of radiolarians, calcareous algae, for- aminifers, sponges, rugose corals, etc, with an extinction rate of 57%. The second episode was located at the base of the conodont zone of Isarcicella isarcica of the Early Triassic, with 71% species going extinct (Song et al., 2013). Moreover, the Paleozoic marine ecosystem lasting for over 200 million years was identified to come to an end and replaced by the modern marine ecosystem at the second episode. Consequently, it is the events of the second episode that caused the more severe biotic crisis and marine ecosystem collapse. It is notable that some faunas were found to show miniaturization prior to the mass extinction (Twitchett, 2007), indicating the presence of stressed oceanic environmental conditions well before the mass extinction (Figure 1).
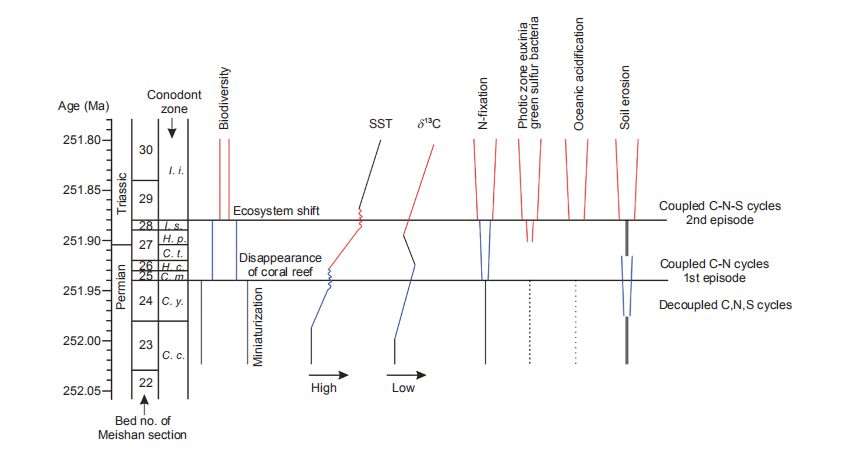
Figure 1 Sketch plot showing the episodic variations of biodiversity, sea surface temperature (SST), biogeochemical cycles of carbon (carbon isotope composition), nitrogen (nitrogen-fixation) and sulfur (photic zone euxinia) and some other marine and terrestrial events across the Permian-Triassic boundary. The blue and the red show the events during the first and second episodes, respectively. Abbreviation for conodont zones (Jiang et al., 2007), C.c., Clarkina changxingensis; C.y., Clarkina yini; C.m., Clarkina meishanensis; H.c, Hindeodus changxingensis; C.t., Clarkina taylorae; H.p, Hindeodus parvus; I.s, Isarcicella staeschei; I.i, Isarcicella isarcica.
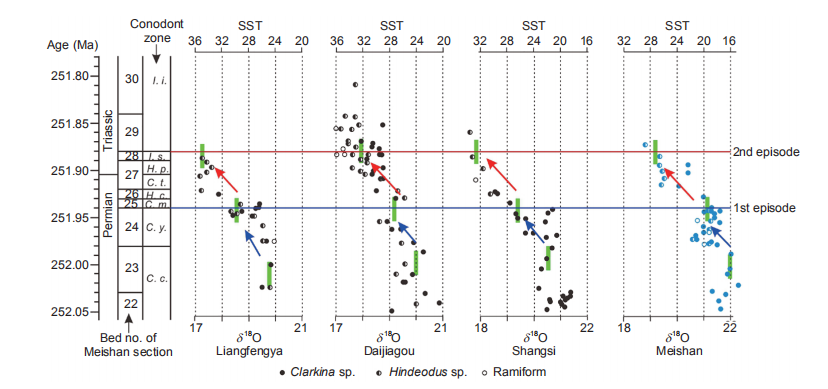
Figure 2 The profiles of oxygen isotope composition (%o) and the calculated sea surface temperature (SST, C) for the 4 sections in tropical South China. The blue and red arrows indicate the rapid rise of the temperature immediately before the 1st and 2nd episodes of faunal mass extinction, and the green lines show the relatively stable trends of the temperature variation (reflected in the oxygen isotope composition within a conodont genus). The data of Meishan are from Joachimski et al. (2012), and the data of the other three sections are from Chen et al. (2016). Please refer to Figure 1 for the abbreviation of the conodont zones.
Associated with the collapse of marine ecosystems is the climate change, in particular the temperature variation (Sun et al., 2012). On the basis of the oxygen isotope composition of the apatite of conodonts measured from 4 sections in South China (Chen et al., 2012, Joachimski et al., 2012), the sea surface temperature (SST) in tropical regions also shows a pattern of two episodic variations (Figures 1 and 2). The first rise in SST was documented to occur at the interval from C. changexingensis to the boundary between C. yini and C. meishanensis at the end-Permian. The SST shifts from 25, 24, 22 and 16 C to 30, 28, 26 and 20 C at Liangfengya and Daijiagou of Chongqing, Shangsi of Sichuan and Meishan of Zhejiang, respectively (Figure 2). The SST variation during this interval is thus about 4-5 C. On the basis of high-re-solution U-Pb dating at Meishan section (Burgess et al., 2014), this sedimentary interval deposited for about 70 ka. It is calculated that the SST variation immediately before the first episode is equivalent to 6-7 C within 100 ka, which is about 3 times larger than the SST variation of Quaternary glacial-interglacial cycles in tropical regions.
The second rise in SST was located at the horizons from the boundary between C. yini and C. meishanensis at the end- Permian to the base of I. isarcica at Early Triassic. The SST shifts ftom 30, 28, 26 and 20 C to 35, 32, 32 and 28 C at Liangfengya and Daijiagou of Chongqing, Shangsi of Sichuan and Meishan ofZhejiang, respectively (Figure 2). The SST variation immediately before the second episode is about 6-7 C within a time duration of about 60 ka, equivalent to a variation of 7-13 C within 100 ka, which is about 36 times larger than the tropical SST variation of Quaternary glacial-interglacial cycles. Consequently, the second episode is featured by the enhanced SST as well as the larger variation of SST (Figure 2) in comparison with the first episode, consistent with the more severe mass extinction and marine ecosystem collapse as discussed earlier.
3.Decoupled biogeochemical cycles prior to the biotic crisis
A global progressive decrease in carbon isotope composition of carbonate was long been observed at the latest Permian in many marine sections (Figure 1). This negative shift indicates the enhanced input of 12C to the surface carbon pool. The 12C added could be sourced from the gas hydrates (Berner, 2002; Krull et al., 2004), or from volcanic activities voluminously releasing gases and extensively burning the underlying Permian coals (Retallack and Jahren, 2008; Svensen et al., 2009; Grasby et al., 2011). Associated with this progressive drop in carbon isotope composition is the miniaturization of some marine faunas observed in conodonts, brachiopods, gastropods, foraminifers and ostracods, inferring the onset of the changes in marine environmental conditions (Figue 1).
However, the nitrogen isotope composition of organic matter does not show any substantial variation below the mass extinction horizons. Similar situation is also observed at the sulfur isotope composition of pyrite or carbonate-associated sulfate. The biogeochemical cycles of carbon, nitrogen and sulfur appears to be in a decoupled pattern well before the faunal mass extinction. This is in particular observed in some shallow water sections in South China (Luo et al., 2011). The decoupled variation of elemental biogeochemical cycles shows the enhanced input of 12C might be too little to change, globally, the nitrogen and sulfur cycles, though it did cause the miniaturization of some marine faunas. This might be indicative of the onset of the changes in some specific marine environmental conditions before the faunal mass extinction.
4.Coupled biogeochemical cycles during faunal mass extinction
The decoupled biogeochemical cycles prior to the mass extinction was immediately followed by a coupled pattern inthe carbon, nitrogen and sulfur cycles during the mass extinction. Carbon isotope composition of carbonate, nitrogen isotope composition of organic matter and sulfur isotope composition of carbonate-associated sulfate all show depletion during the faunal mass extinction (Luo et al., 2010, 2011). It is further noteworthy that the coupled interval is associated with the enhanced global warming.
The continuous negative shift of carbon isotope composition at the latest Permian indicates the elevated input of 12C to the surface systems, much greater than that prior to the biotic crisis. The nitrogen isotope composition of organic matter was observed to drop to 0-1%o, inferring the contemporaneous enhancement of nitrogen-fixation (Luo et al., 2011; Jia et al., 2012). This enhancement is further supported by the elevated values in the 2-methyhopane index, which is indicative of the bloom of nitrogen-fixation bacteria in many marine sections, including those in the deep water facies such as the Shangsi section in South China. In Bulla section in Italy, a negative correlation between the 2-methyhopane index and the nitrogen isotope composition of organic matter was only observed during the faunal mass extinction (Jia et al., 2012). It appears that the enhanced 12C input is coupled with the enhanced nitrogen fixation during the biotic crisis. The Shangsi section indicates the nitrogen-fixation is much stronger during the second episode of the faunal mass extinction (Xie et al., 2017b).
Associated with the coupled C-N processes is the decrease of sulfur isotope composition, which might be indicative of the small pool of oceanic sulfate during the faunal mass extinction (Luo et al., 2010). It is notable, however, the abundances of aryl isoprenoid and isorenieratane, the biomarkers of green sulfur bacteria, indicate that photic zone euxinia was only found during the second period but absent during the first episode as shown at Shangsi section in South China (Xie et al., 2017b). It is further demonstrated that photic zone euxinia might be only present in some local sites during the first episode but clearly expand to a broad region or even to the globe during the second episode (Zhou et al., 2017). It appears the first episode is mainly featured by the coupled C-N biogeochemical cycles whilst the second episode is characterized by the coupled C-N-S biogeochemical cycles. Consequently, a shift in the coupled biogeochemical cycles from C-N to C-N-S was present when the faunal mass extinction proceeded from the first episode to the second one (Figure 1), showing the importance of microbial activities to feedback the changes in Earth environments (Xie et al., 2016).
These coupled biogeochemical cycles were observed in the intervals featured by the global warming as shown by the SST calculated on the basis of oxygen isotope composition of conodonts as discussed earlier. Their association indicates the input of 12C, the enhanced nitrogen-fixation, photic zone euxinia, small oceanic sulfate pool and the global warming might be connected with each other during the faunal mass extinction. In detail, the input of 12C to Earth surface system might lead to the global warming and the enhanced oceanic stratification. Enhanced oceanic stratification as shown by the elevated values of gammaceranes in some sections led to the occurrence of anoxic condition, which in turn promotes the nitrogen-fixation. These processes caused the coupled C- N biogeochemical cycles as observed during the first episode of the faunal mass extinction. These C-N coupled processes occurring during the first episode could further develop to cause the occurrence of photic zone euxinia, which led to the coupled C-N-S processes observed during the second episode. The coupled C-N-S processes were shown by the maxima of the molecular and isotopic data during the second episode of the faunal mass extinction (Xie et al., 2017b), including the oceanic stratification, nitrogen-fixation and photic zone euxinia. The C-N-S biogeochemical cycles were associated with the remarkably enhanced global warming during the second episode, inferring their causal relationship. All these processes indicate the presence of the more deteriorated marine environmental conditions during the second episode which further explains the more severe mass extinction and the shift of the marine ecosystem occurring during that period. Consequently, the biogeochemical cycle shows a shift from coupled C-N processes at the first episode to coupled C-N-S processes at the second episode, when the global warming is much enhanced, and they both lead to the more mortality in marine ecosystems (Figure 1).
5. Implication for the modern oceans
The shifts of biogeochemical cycles, associated with the faunal mass extinction and the global warming across the Permian-Triassic boundary about 252 million years ago, provide the analog to understanding the modern oceans.
First, along with the progressive global warming, the biogeochemical cycle shows a shift from the decoupled processes of carbon, nitrogen and sulfur prior to the mass extinction to the coupled C-N processes during the first episode of faunal mass extinction, and finally to the coupled C-N-S processes during the second episode. The biogeochemical cycles could thus be an indication to the progressive collapse of marine ecosystems triggered by the global warming in both the ancient times and the modern days.
Second, prior to the faunal mass extinction, the biogeochemical cycles of carbon, nitrogen and sulfur were identified to show a decoupled pattern. Enhanced input of 12C, probably in a small amount, appears not to impact the global biogeochemical cycles of nitrogen and sulfur during that period, indicating the marine environmental conditions are not very deteriorated. However, the miniaturization of some marine faunas is present, in association with the enhanced input of12C. This could be an early warning ofthe collapse of marine ecosystems.
Third, the shift of the coupled biogeochemical cycles from C-N to C-N-S when the mass extinction proceeded from the first episode to the more severe second episode indicates the progressive development of the more deteriorated marine environmental conditions. In modern oceans, the coupled C- N cycle was observed in some regions, i.e., the enhanced input of 12C and the global warming have led to the enhancement of the nitrogen-fixation (Ulloa et al., 2012). If these local C-N processes continue to develop and expand to the globe, the coupled C-N-S processes might be brought into existence and the marine ecosystems are inevitable to go into complete collapse as observed 252 million years ago. In some marine regions, cryptic sulfur cycle was observed (Canfield et al., 2010), inferring that the C-N-S coupled processes were occasionally observed, which raises our great attention to the causes of these processes.
Acknowledgements The work was supported by the State Key R&D Project (Grant No. 2016YFA0601100), the National Natural Science Foundation of China (Grant No. 41330103) and the 111 Project of China (Grant No. B08030).
References
Barnosky A D, Matzke N, Tomiya S, Wogan G O U, Swartz B, Quental T B, Marshall C, McGuire J L, Lindsey E L, Maguire K C, Mersey B, Ferrer E A. 2011. Has the Earth's sixth mass extinction already arrived? Nature, 471: 51-57
Berner R A. 2002. Examination of hypotheses for the Permo-Triassic boundary extinction by carbon cycle modeling. Proc Natl Acad Sci USA, 99: 4172-4177
Burgess S D, Bowring S, Shen S. 2014. High-precision timeline for Earth's most severe extinction. Proc Natl Acad Sci USA, 111: 3316-3321
Canfield D E, Stewart F J, Thamdrup B, De Brabandere L, Dalsgaard T, Delong E F, Revsbech N P, Ulloa O. 2010. A cryptic sulfur cycle in oxygen-minimum-zone waters off the Chilean coast. Science, 330: 1375-1378
Chen J, Shen S, Li X, Xu Y, Joachimski M M, Bowring S A, Erwin D H, Yuan D, Chen B, Zhang H, Wang Y, Cao C, Zheng Q, Mu L. 2016. High-resolution SIMS oxygen isotope analysis on conodont apatite from South China and implications for the end-Permian mass extinction. Palaeogeogr Palaeoclimatol Palaeoecol, 448: 26-38
Erwin D H. 2006. Extinction: How life on Earth nearly ended 250 million years ago. Biologist, 311: 1868-1869
Grasby S E, Sanei H, Beauchamp B. 2011. Catastrophic dispersion of coal fly ash into oceans during the latest Permian extinction. Nat Geosci, 4: 104-107
Jia C, Huang J, Kershaw S, Luo G, Farabegoli E, Perri M C, Chen L, Bai X, Xie S. 2012. Microbial response to limited nutrients in shallow water immediately after the end-Permian mass extinction. Geobiology, 10: 60-71
Jiang H, Lai X, Luo G, Aldridge R, Zhang K, Wignall P. 2007. Restudy of conodont zonation and evolution across the P/T boundary at Meishan section, Changxing, Zhejiang, China. Glob Planet Change, 55: 39-55
Jin Y G, Wang Y, Wang W, Shang Q H, Cao C Q, Erwin D H. 2000. Pattern of marine mass extinction near the Permian-Triassic boundary in South China. Science, 289: 432-436
Joachimski M M, Lai X, Shen S, Jiang H, Luo G, Chen B, Chen J, Sun Y. 2012. Climate warming in the latest Permian and the Permian-Triassic mass extinction. Geology, 40: 195-198
Krull E S, Lehrmann D J, Druke D, Kessel B, Yu Y Y, Li R. 2004. Stable carbon isotope stratigraphy across the Permian-Triassic boundary in shallow marine carbonate platforms, Nanpanjiang Basin, south China. Palaeogeogr Palaeoclimatol Palaeoecol, 204: 297-315
Luo G, Kump L R, Wang Y, Tong J, Arthur M A, Yang H, Huang J, Yin H, Xie S. 2010. Isotopic evidence for an anomalously low oceanic sulfate concentration following end-Permian mass extinction. Earth Planet Sci Lett, 300: 101-111
Luo G, Wang Y, Algeo T J, Kump L R, Bai X, Yang H, Yao L, Xie S. 2011. Enhanced nitrogen fixation in the immediate aftermath of the latest Permian marine mass extinction. Geology, 39: 647-650
Retallack G J, Jahren A H. 2008. Methane release from igneous intrusion of coal during Late Permian extinction events. J Geol, 116: 1-20
Song H, Wignall P B, Tong J, Yin H. 2013. Two pulses of extinction during the Permian-Triassic crisis. Nat Geosci, 6: 52-56
Sun Y D, Joachimski M M, Wignall P B, Yan C, Chen Y, Jiang H, Wang L, Lai X. 2012. Lethally hot temperatures during the Early Triassic greenhouse. Science, 338: 366-370
Svensen H, Planke S, Polozov A G, Schmidbauer N, Corfu F, Podladchikov Y Y, Jamtveit B. 2009. Siberian gas venting and the end-Permian environmental crisis. Earth Planet Sci Lett, 277: 490-500
Twitchett R J. 2007. The Lilliput effect in the aftermath of the end-Permian extinction event. Palaeogeogr Palaeoclimatol Palaeoecol, 252: 132-144
Ulloa O, Canfield D E, DeLong E F, Letelier R M, Stewart F J. 2012. Microbial oceanography of anoxic oxygen minimum zones. Proc Natl
Zhou W, Algeo T J, Ruan X, Luo G, Chen Z Q, Xie S. 2017. Expansion of photic-zone euxinia during the Permian-Triassic biotic crisis and its causes: Microbial biomarker records. Palaeogeogr Palaeoclimatol Pa- laeoecol, 474:
Acad Sci USA, 109: 15996-16003
Xie S, Pancost R D, Yin H, Wang H, Evershed R P. 2005. Two episodes of microbial change coupled with Permo/Triassic faunal mass extinction. Nature, 434: 494-497
Xie S, Pancost R D, Huang J, Wignall P B, Yu J, Tang X, Chen L, Huang X, Lai X. 2007b. Changes in the global carbon cycle occurred as two episodes during the Permian-Triassic crisis. Geology, 35: 1083-1086
Xie S C, Yin H F. 2014. Progress and perspective on frontiers of geobiology. Sci China Earth Sci, 57: 855-868
Xie S C, Liu D, Qiu X, Huang X Y, Algeo T J. 2016. Microbial roles equivalent to geological agents ofhigh temperature and pressure in deep Earth. Sci China Earth Sci, 59: 2098-2104
Xie S C, Chen J F, Wang F P, Xun L Y, Tang K, Zhai W D, Liu J H, Ma W T. 2017a. Mechanisms of carbon storage and the coupled carbon, nitrogen and sulfur cycles in regional seas in response to global change. Sci China Earth Sci, 60: 1010-1014
Xie S, Algeo T J, Zhou W, Ruan X, Luo G, Huang J, Yan J. 2017b. Contrasting microbial community changes during mass extinctions at the Middle/Late Permian and Permian/Triassic boundaries. Earth Planet Sci Lett, 460: 180-191
Yin H, Xie S, Luo G, Algeo T J, Zhang K. 2012. Two episodes of environmental change at the Permian-Triassic boundary of the GSSP section Meishan. Earth-Sci Rev, 115: 163-172