Blue carbon on the rise: challenges and opportunities
Nianzhi Jiao1, Hong Wang2, Guanhua Xu3 and Salvatore Aric`o4
BLUECARBONONTHERISE
Climate change is a global concern that requires urgent solutions. As a signatory to the Paris Agreement, China has committed to have its greenhouse gas emission reach a peakby the year 2030, which means that severe countermeasures for reducing emissions have to be put into practice. This is a hard mission given that development is still the top priority in the years to come for China. Under such circumstances, enhancing carbon sequestration becomes an effective approach to achieving the goal. While terrestrial green carbon sink is already in practice, the ocean carbon reservoir, containing 93% of global CO2, as 50 and 20 times the carbon inventories of atmosphere and land, respectively, has great potential to expand. Each year, at least 25% of the anthropogenic CO2 has been captured by marine ecosystems as blue carbon [1].
In 2009, the United Nations Environment Programme (UNEP), the Food and Agricultural Organization of the United Nations (FAO) and the Intergovernmental Oceanographic Commission (IOC) of the United Nations Educational, Scientific and Cultural Organization (UNESCO) jointly published the Blue Carbon report, pointing out that more than 55% of global primary production is blue carbon [2]. Moreover, the production efficiency of the coastal blue carbon biomes (mangroves, sea grass, salt marsh ecosystems, etc.) is much higher than that of the Amazon rain forest. However, these blue carbon biomes are being degraded and disappearing at rates 5-10 times faster than rainforests [3], thus protection and restoration are in urgent need.
Besides the visible coastal blue carbon, there are enormous invisible blue carbon biomes composed of tiny but extremely abundant microbes, including phytoplankton, bacteria, archaea and viruses, which contribute up to 95% of the world's blue carbon [4-6]. These microbes can interact with the visible blue carbon biomes, transforming their organic carbon into refractory forms, prolonging the residence time of the organic carbon in the ocean. The environmental issues we are facing in the coastal water today (such as eutrophication,hypoxia, acidification, etc.) also interact with the invisible blue carbon processes, but the mechanisms are not yet clear. Therefore, both visible and invisible blue carbon biomes should be taken into consideration for marine ecosystem services, and understanding their interactions and their relationship with environmental issues is critical for marine ecosystem management and sustainable development.
BLUE CARBONINITIATIVEAND PROGRESS
The IOC, Conservation International (CI) and the International Union for Conservation of Nature (IUCN) jointly launched the Blue Carbon Initiative in 2011, aimed at promoting the management of marine and coastal ecosystems through international cooperation, and maintaining carbon sink function in the mitigation of climate change. ‘Blue Carbon Action' set up two working groups. One is the scientific group toward the establishment of blue carbon measurement and monitoring protocols, data acquisition and quality control, field survey handbooks as well as blue carbon conservation planning and management guidelines. The other is the policy working group toward integration of the blue carbon project into the United Nations Framework Convention on Climate Change and Convention on Biological Diversity, and the development of a program of financial support and other policies. Since then, ‘Blue Carbon Action' has been in action in the protection and restoration of mangroves, wetlands, etc. in many regions and countries such as Australia, Dhabi, Indonesia, India, Kenya, Madagascar, Vietnam and the USA.
Significant progress has been made in coastal blue carbon research in recent years, such as the description ofblue carbon status and carbon sequestration potentials at the global scale [7,8] and regional scale [8]; the assessment of impacts of wetland destruction and the proposal of corresponding policy [7]; the evaluation of the eco-value of blue carbon in sea grasses, salt marshes and mangroves (e.g. in Philip Bay and Western Harbor, Australia, with an estimate of the rooted plants to be 1.03 million tons of carbon and apriceof $15.38 million) [9]; proposal of countermeasures for conservation and restoration of blue carbon (e.g. in Scotland, Columbia, Korea and other countries) [10,11]; as well as scenario simulation of blue carbon benefit function and market price [12]. In 2017, the Intergovernmental Panel on Climate Change (IPCC) launched the writing process of the Sixth Assessment Report on Climate Change, with a Special Report on the Ocean and Cryosphere in which blue carbon is included.
CHALLENGES AND OPPORTUNITIES
Although the original concept of blue carbon proposed in 2009 refers to the carbon that is captured by marine ecosystems covering both coastal and open waters [3], practical research and development of blue carbon have predominantly involved macrofauna, such as mangrove, seagrass and salt marsh in the coastal zone. After years of promotion of the coastal blue carbon, the IUCN published the Call for Action on Ocean- Carbon in 2014, which highlights the importance of ocean carbon sinks in the mitigation of climate change, and identifies the key components of blue carbon in open oceans, expanding the blue carbon from coastal zones to oceanic environments [13]. In fact, the invisible microbes are an essential part ofblue carbon in the ocean, but have been largely overlooked so far. These microbes, including phytoplankton (microalgae), cyanobacteria, bacteria, archaea and viruses, are tiny but extremely abundant, contributing up to 90% of the marine biomass and 95% of the marine production [4-6]. Annually, over 360 billion tons of CO2 is fixed by marine phytoplankton, 1.39% of which is transported down to the seafloor by the biological pump (BP) for longterm storage [14]. The rest of the fixed organic carbon is mainly respired into CO2 , but a small portion of the organic carbon is shunted through the microbial carbon pump (MCP) to biologically inaccessible phases, being either refractory or at extremely low concentrations [15]. The MCP is a major contributor to the tremendous marine refractory DOC (RDOC) reservoir, which isequivalent in amount to the total inventory of CO2 in the atmosphere [16]. Paleoclimate studies show that there was an inextricable link between the RDOC pool and climate change [17,18]. The MCP effects exist in all water environments and even soil environments [19], connecting with the visible blue carbon ecosystem, as all the blue carbon macro-biomes (mangrove, sea grass, salt marsh, etc.) release DOC into the water, which can be further transformed by the MCP into RDOC. Such processes are influenced by environmental conditions and thus allow manipulations to pursue maximum outputs of the sum of the BP and MCP (Fig. 1).
LAND-SEAINTEGRATED COUNTERMEASURES FOR CARBON SEQUESTRATION AND SUSTAINABLE DEVELOPMENT OFTHE COASTAL ECOSYSTEM
Chemical fertilizers have been excessively applied in farming for decades, especially in developing countries. Excessive nitrogen (N) and phosphorus (P) are then washed out into rivers and ultimately discharged into the coastal waters, causing eutrophication and algal blooms. Although algal blooms seemingly produce more organic carbon, this carbon is basically labile and can be respired rapidly. In addition, the labile DOC produced by primary producers has priming effects on the river discharged terrestrial RDOC, namely remobilizing RDOC for microbial uptake and respiration, which can create high CO2 concentrations in the water, making the carbonate equilibrium system move toward proton generation causing acidification in ambient water, and excess CO2 escape from water to atmosphere as outgassing. That is why productive estuarine and coastal waters are often sources rather than sinks of atmospheric CO2. Meanwhile, this process consumes a large quantity of oxygen, resulting in hypoxia. Anoxic conditions could cause massive death of macro- and microbiomes, resulting in the breeding of anaerobic bacteria that transform organics into CH4, H2S, N2O and other toxic substances, which in turn are destructive for the ecosystem. On top of that, excess discharge of nutrients (N, P) shapes the C/N and C/P elemental ratios in favor of remobilization of RDOC for respiration, lowering the MCP efficiency and carbon sequestration. Therefore, reducing terrestrial input of inorganic nutrients becomes a feasible countermeasure for the enhancement of carbon sequestration in coastal waters (Fig. 1). This idea is supported by a statistical data analysis of organic carbon versus nitrate in various natural environments [20] as well as by experimental results in estuarine and offshore waters, which found that, in all cases, if there are too many inorganic nutrients, there will be less organic carbon preserved in the environment. Therefore, land-ocean integrated management and engineering become necessary, such as reducing the application ofchemical fertilizers in farming and eliminating sewage discharge into rivers so as to reduce the N, P inputs into the sea. Such eco-engineering is not aimed at changing the natural ecosystems, but rather protecting them by reducing eutrophication and red-tides occurrence while increasing carbon sequestration through the MCP. This idea also brings new policy as bonus-based carbon trade rather than penalty-based pollution policy, as is being used in many countries nowadays. The bonusbased policy would be such that any economy loss claimed due to the reduction of farming fertilization (should not be the case if fertilization is scientifically applied, though) and sewage work in the watersheds can be compensated by the eco-value or carbon price of the increment of carbon sequestration in the sea. Once a carbon-accounting system for the watershed-coastal-offshore environments is established, a blue carbon sequestration-based voluntary emission reduction trading mechanism could be easily developed.
BLUECARBON STRATEGY IN CHINA
The China Seas include the Bohai Sea, the Yellow Sea, the East China Sea and the South China Sea, with coastlines of 18 000 km, stretching from the northern temperate zone to tropic zones. There are more than 1500 rivers to the China Seas, including the world third largest river, the Yangtze River to the East China Sea, the Yellow River carrying a huge amount of sediment to the Bohai Sea and the Pearl River to the South China Sea connecting the Tibetan plateau with the ‘warm pool' in the West Pacific. Such rich habitats harbor great biodiversity and carbon-storage capacity. On the other hand, China's coastal zones are also highly inhabited, with most of them receiving severe anthropogenic impacts such as harbor construction, tidal zone reclamation, as well as mariculture. Discharges of nutrients and organic matter from rivers as well as sea-farming activities have fundamental impacts on the ecosystem's health and sustainability. Many of the estuarine waters are currently suffering from algal bloom, hypoxia and acidification caused by eutrophication and the following microbial processes as pointed out in the ‘Challenges and Opportunities' section above.
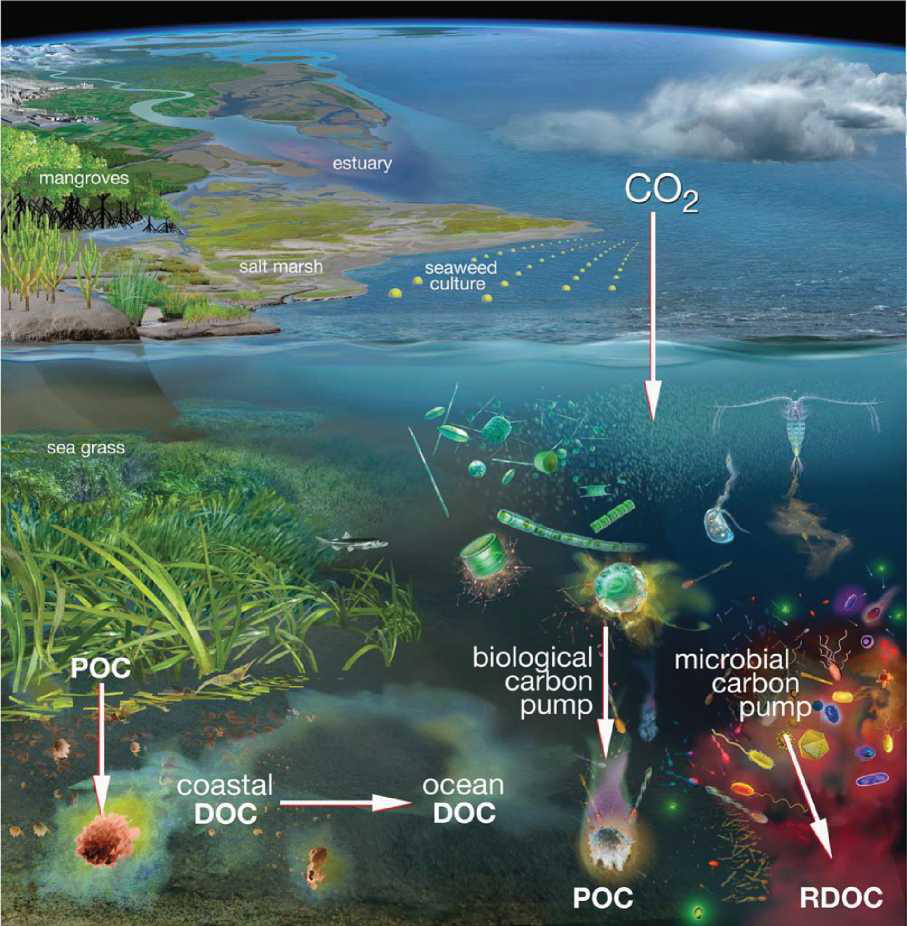
Figure 1. A demo of eco-engineering to make coastal waters into sinks rather than sources of atmospheric CO2. In addition to the conservation and restoration of coastal blue carbon (mangroves, salt marshes, sea grasses, etc.), reducing terrestrial nutrient inputs could avoid the priming effects on organic carbon respiration and thus increase carbon sequestration in the ocean through both the BP and the MCP.
Despite coastal rooted plants like mangroves, saltmarshes and sea grasses having high carbon production, their coverage in China's coastal areas is small and the annual buried amounts of organic matter from them are limited (Table 1). Meanwhile, their production of DOC has never been counted due to lack of study. Take the case of DOC release from cultured seaweeds, for example (although cultivated seaweeds are not counted as blue carbon officially, its buried debris and derived RDOC do contribute to marine carbon sink). The total production of China's seaweeds culture is about 3.52 Tg C yr-1. If 23-26% of this carbon is released as DOC [21], and assuming that only half of the DOC becomes refractory (in the water column, 77-94% of the bulk DOC is refractory [22]), a conservative estimate of the RDOC derived from seaweed culture would be 0.5 Tg C yr-1 [22], which is even higher than the total burial flux of organic carbon from the coastal blue carbon in China.
When looking at blue carbon on larger scales, terrestrial inputs of DOC to the coastal waters and marginal sea export of DOC to oceanic waters become essential for the mitigation of climate change. Each year, a great deal of DOC is discharged into China's seas by rivers. For example, the annual DOC flux of the Yangtze River to the East China Sea is 1.62 Tg C yr-1 [22], and the annual export flux of DOC from the East China Sea to the Western Pacific is 15-35 TgC yr-1 [23]. Such DOC has been exposed to a variety of environmental conditions before it is exported and is supposed to be relatively refractory. If it keeps the recalcitrance under controlled nutrient inputs (as discussed in the above section) instead of being remobilized under eutrophic conditions and respired in the marine environments, it would be a substantial contribution to marine carbon sequestration [24]. Together with the BP flux on the continental shelf (7.4 Tg C yr-1) [22], the East China Sea is actually a significant carbon sink. In fact, the total carbon export flux of China's continental shelves is up to 90 Tg C yr-1 (Table 1); this recognition changes the overall image of China's seas as ‘sources' as perceived from the exchange of CO2 between the atmosphere and sea surface. Such ‘sources' are actually due to the respiration of terrestrial DOC and the outgassing of imported high-dissolved inorganic carbon (DIC) deep water from the Western Pacific [22]. It is therefore worth pointing out that, even if a marine region is the source of atmospheric CO2, carbon sequestration could take place at the same time, justlikethe case of oceanicup- welling areas.
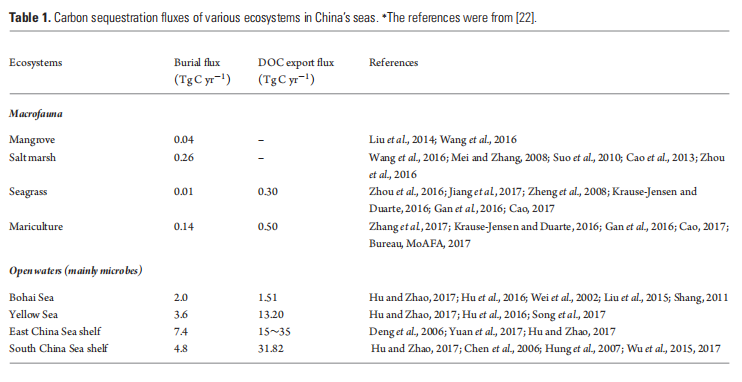
Based on the above understanding, an effective strategy for China's blue carbon project would be that, while deploying restoration of the visible coastal blue carbon (rooted plants), efforts should be made in invisible microbial carbon sequestration in the waters, especially estuarine and shelf regions. Only in that case will the blue carbon project become essentially meaningful for the mitigation of climate change. In practice, the following measures should be implemented: to establish long-term monitoring and observation networks covering representative sites in the river catchments, estuaries as well as shelf waters; to establish standard protocols for core measurements of different forms of blue carbon in various ecosystems; to establish the mechanisms of blue carbon eco-value assessment; to establish a carbon-accounting system for the watershed-coastal-offshore environments; to establish a land-ocean integrated compensation policy to promote blue carbon trading with the farming industry; to establish a framework for voluntary emission reduction trading with blue carbon for low carbon economy.
ACKNOWLEDGEMENTS
We thank the participants of the 1st Yanqi Lake conference and the PICES-ICES WG on Biological Driven Carbon Pumps for their discussions and comments; Glynn Gorick and Tingwei Luo for their help with the figure. This work was supported by the National Key Research Program of China (2016YFA0601400), the State Oceanic Administration project (GASI-03-01-02-05), the National Natural Science Foundation of China(91751207), the Chinese Academy of Sciences consulting program (2016ZWH008A-008), the NSFC-CAS discipline strategy program (L1624030) and the Fundamental Research Funds for the Central Universities (20720170107).
Nianzhi Jiao1,*, Hong Wang2, Guanhua Xu3 and Salvatore Arico4
1 State Key Laboratory of Marine Environmental Science, Xiamen University, China 2State Ocean Administration, China ‘Ministry of Science and Technology, China 4Intergovernmental Oceanographic Commission of United Nations Educational, Scientific and Cultural Organization (UNESCO), France
* Corresponding author.
E-mail: jiao@xmu.edu.cn
REFERENCES
1.Le Quere C, Moriarty R and Andrew RM et al. Earth Syst Sci Data 2015; 7: 47-85.
2.Bowler C, Karl DM and Colwell RR. Nature2009; 459: 180-4.
3.Nellemann C, Corcoran E and Duarte CM et al. Blue Carbon: The Role of Healthy Oceans in Binding Carbon: A Rapid Response Assessment. GRID-Arendal: United Nations Environment Programme, 2009.
4.Sogin ML, Morrison HG and Huber JA et al. Proc Natl Acad Sci USA 2006; 103: 12115-20.
5.Suttle CA. Nat Rev Micro 2007; 5: 801-12.
6.Pomeroy LR, Williams PJI and Azam F et al. Oceanog 2007; 20: 28-33.
7.Sifleet S, Pendleton L and Murray BC. Nicholas Institute Report 2011; 11: 06.
8.Pendleton L, Donato DC and Murray BC etal. PLoS One 2012; 7: e43542.
9.Carnell P, Ewers C and Rochelmeyer E et al. The Distribution and Abundance of 'Blue Carbon' within Port Phillip and Westernport. Melbourne: Deakin University, 2015.
10.Burrows MT, Kamenos NA and Hughes DJ et al. Assessment of Carbon Budgets and Potential Blue Carbon Stores in Scotland's Coastaland Marine Environment. Edinburgh: Scottish National Heritage, 2014.
11.SondakCFAand Chung IK. Ocean Sci J2015; 50: 1-8.
12.Zarate-Barrera TG and Maldonado JH. PLoSOne 2015; 10: e0126627.
13.Howard J, Sutton-GrierAand Herr D etal. Front Ecol Environ 2017; 15: 42-50.
14.Katherina S, Christian H and Matthias Z. Global Biogeochem Cycles 2005; 19: 155-72.
15.Jiao N, Herndl GJ and Hansell DA et al. Nat Rev Micro 2010; 8: 593-9.
16.Hansell DA, Carlson CA and Repeta DJ et al. Oceanog 2009; 22: 202-11.
17.Rothman DH, Hayes JM and Summons RE. Proc Natl Acad Sci USA 2003; 100: 8124-9.
18.Ridgwell A. Proc Natl Acad Sci USA 2011; 108: 16485-6.
19.Liang C, Schimel JP and Jastrow JD. Nat Microbiol 2017; 2: 17105.
20.Taylor PG and Townsend AR. Nature 2010; 464: 1178-81.
21.Krause-Jensen D and Duarte CM. Nature Geosci 2016; 9: 737-42.
22.Jiao N, Liang Y and Zhang Y etal. Comprehensive analysis of carbon pool and fluxes in China Sea and its adjacent oceans. Sci China Earth Sci 2018; doi:10.1007/s11430-018-9190-x.
23.Yuan D, He J and Li J et al. Sci China Earth Sci 2018; 61: 659-67.
24.Jiao N, Robinson C and Azam F et al. Biogeosciences 2014; 11: 5285-306.
National Science Review
5: 464-468, 2018 doi: 10.1093/nsr/nwy030
Advance access publication 15 February 2018